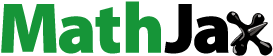
ABSTRACT
The aim of this work is to study the effect of eggshell powder (ESP) on the properties of corn starch (CS) films. It was found that the addition of ESP significantly improved the tensile strength, elongation at break, water vapor and oxygen barrier properties compared to the control film. There was an inductive effect between the C–C bonds on cornstarch skeleton and the O–C–O bond on CaCO3 in the ESP and the number of hydrogen bands increased between starch molecules and ESP particles, which indicated a strong interaction and biocompatibility between the two components. In addition, the organic compounds on the surface of ESP increased the adhesion between CaCO3 particles and CS films matrix. In summary, the ESP, as an enhancement factor, is a good candidate for the development of green food packaging material.
Graphical Abstract
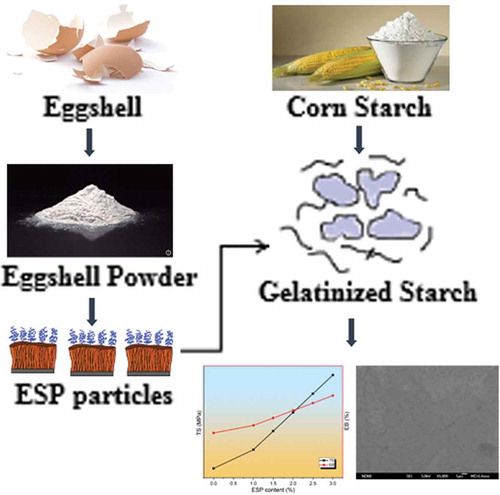
RESUMEN
El presente trabajo se propuso estudiar el efecto del polvo de la cáscara de huevo (ESP) en las propiedades de películas de almidón de maíz (CS). Se comprobó que, en comparación con la película de control, la adición de ESP mejora significativamente la fuerza tensil, el alargamiento a la rotura y las propiedades de barrera contra el vapor de agua y el oxígeno. Asimismo, se estableció que existe un efecto inductivo entre los enlaces C–C en el esqueleto de almidón de maíz y el enlace O–C–O en el CaCO3 de la ESP. Además, se constató el aumento del número de bandas de hidrógeno entre las moléculas de almidón y las partículas de la ESP, lo cual da cuenta de la existencia de una fuerte interacción y biocompatibilidad entre los dos componentes. Por otra parte, los compuestos orgánicos presentes en la superficie de la ESP aumentaron la adhesión entre las partículas de CaCO3 y la matriz de películas de CS. En resumen, en tanto factor de intensificación, la ESP constituye una buena opción para desarrollar materiales ecológicos destinados al envase de alimentos.
1. Introduction
Bio-based polymers, a new type of materials with green, environmentally friendly, renewable and biodegradable properties, are manufactured using renewable biomass through biological, physical or chemical methods (Felix, Perez-Puyana, Romero, & Guerrero, Citation2017; Lambert & Wagner, Citation2017). In accordance with the sources of raw materials, bio-based polymer materials including natural polymers such as polysaccharide and synthetic polymers such as poly(lactic acid) (Xiaofei Ma, Lv, Anderson, & Chang, Citation2017; Shi, Jing, Zhang, Xu, & Yao, Citation2017; Yuan et al., Citation2016). These bio-based materials can partially replace the traditional petroleum-based plastics and can ease the oil crisis and reduce environmental pollution, exhibited a broad market space and application prospect (Liu et al., Citation2017; Marcet, Saez, Rendueles, & Diaz, Citation2017).
Starch, as a natural polymer material, is composed of D-glucose linked by the glycosidic bonds (Luchese, Spada, & Tessaro, Citation2017). It is widely used in food, chemical, textile and building materials industries, and is also one of the most promising materials for applications in food packaging (Piñeros-Hernandez, Medina-Jaramillo, López-Córdoba, & Goyanes, Citation2017; Saberi et al., Citation2016). As a potential alternative to conventional plastics, several novel bio-based materials have been investigated from corn (Li et al., Citation2018), wheat (Song, Zuo, & Chen, Citation2018), cassava (Edhirej, Sapuan, Jawaid, & Zahari, Citation2017), potato (Malmir et al., Citation2018), pea (Saberi et al., Citation2017), and sugar palm starch ((Jumaidin, Sapuan, Jawaid, Ishak, & Sahari, Citation2016; Sahari, Sapuan, Zainudin, & Maleque, Citation2013; Sanyang, Sapuan, Jawaid, Ishak, & Sahari, Citation2015). Cornstarch (CS) has attracted exclusive attention for the preparation of biodegradable films because of its low cost and the largest source (Chen et al., Citation2017). In addition, cornstarch has good film-forming properties due to its high amylose content (Wang et al., Citation2017). However, CS-based plastics exhibit several drawbacks compared to other polymers, including poor mechanical properties and limited stability caused by water absorption (Chen, Shan, Woo, & Chen, Citation2017; Moreno, Cardenas, Atares, & Chiralt, Citation2017). In recent years, the incorporation of nanoparticles to the film matrix to improve the barrier and mechanical properties has drawn close attention of researchers (Chaichi, Hashemi, Badii, & Mohammadi, Citation2017; Miranda et al., Citation2015). Nano-TiO2,(Oleyaei, Zahedi, Ghanbarzadeh, & Moayedi, Citation2016) montmorillonoid,(Romero-Bastida et al., Citation2016) nanofibers (H. Sun, Shao, & Ma, Citation2016), and starch nanocrystals (Bel Haaj, Thielemans, Magnin, & Boufi, Citation2016) have been widely used in the field of nanocomposite materials. The addition of these nanoparticles effectively improved the mechanical properties, barrier properties, and thermal stability of the composite films (Xiaohan Ma et al., Citation2017; Niazi, Jahan, Berg, & Gregersen, Citation2017; Souza, Niehues, & Quadri, Citation2016). However, the elongation at break of the films could be decreased due to the larger rigid structure of the most nanoparticles and the hydrophobic characteristics of some inorganic particles (Oleyaei, Almasi, Ghanbarzadeh, & Moayedi, Citation2016; Oleyaei et al., Citation2016). Therefore, it is particularly important to seek for a bio-filler that can increase the elongation at break under the premise of enhancing the mechanical strength of the films (Shafiur Rahman, Aftab, Shariful Islam, Mukhlish, & Ali, Citation2016).
The eggshell, a highly ordered porous ceramic, contains about 95% calcium carbonate and 5% organic materials such as collagen, sulfated polysaccharides, and other proteins. (Ji, Zhu, Qi, & Zeng, Citation2009; Kang, Pal, Park, Bang, & Kim, Citation2010; Z. Liu, Song, Zhang, He, & Linhardt, Citation2017). With the large consumption of eggs, the amount of eggshell wastes also increased significantly and the weight accounts for 11% of the total weight of eggs (Murugan, Munusamy, & Ismail, Citation2017; Park et al., Citation2016). Therefore, the rational utilization of waste eggshell is conducive to environmental protection (King’ori, Citation2011). The eggshell powder (ESP), a natural calcium source and nutrient enhancer, prepared from eggshells through the cleaning, sterilization and crushing process (Bashir, Manusamy, Chew, Ismail, & Ramasamy, Citation2017; Witoon, Citation2011). The industrial application of ESP has been investigated as an additive for the flour to improve the strength and toughness of gluten, as a calcium supplement for the feed, as accessories for the daily cosmetics, and as soil amendment for the immobilization of cadmium and lead (Ok et al., Citation2011; Quina, Soares, & Quinta-Ferreira, Citation2017; Veloso Naves, Citation2007). In addition, the ESP has also been regarded as a good bio-filler for preparing polymer composites due to its abundance, low price, and renewable resources (Buakaew, Ruksakulpiwat, Suppakarn, & Sutapun, Citation2013; Prabhakar, Rehaman Shah, & Song, Citation2016). Ji et al. investigated the effect of the ESP on the properties of the epoxy resins. They found that the eggshell microparticles have much large specific surface area than commercial calcium carbonate and the toughness of epoxy resin composite could be improved by the incorporation of ESP even without the addition of coupling agent (Ji et al., Citation2009). Munlika et al. prepared the thermoplastic starch/eggshell powder composites by compression molding. They found that the ESP improved the thermal stability of thermoplastic starch (TPS), and the TPS/ESP composites showed a faster degradation speed and a lower water absorption compared to the TPS/calcium carbonate composites (Bootklad & Kaewtatip, Citation2013).
Considering the composition and special structure of eggshell powder (ESP), the main objective of this paper was to develop the cornstarch (CS)-based composite films reinforced with ESP by solution casting method and evaluate the effects of ESP concentration on the mechanical properties, barrier properties, thermal stability and biodegradation of films. The particle size, morphology, and structure of ESP were measured by particle size analyzer, scanning electron microscope (SEM), Fourier transform infrared spectroscopy (FTIR), and X-ray diffraction (XRD). In order to disperse the ESP uniformly in the CS matrix, put it in a mortar and ground with a small amount of distilled water in advance, and then the suspension is stirred by magnetic stirrer for 2 h to make the ESP particles wet completely. The results show that the ESP increased the tensile strength (TS) and elongation at break (EB) of composite films, and the barrier properties and thermal stability also were improved. This confirmed that the ESP as a bio-filler has great potential to improve the toughness of composite films in the field of food packaging.
2. Experimental
2.1. Materials
Native corn starch (CS) was kindly supplied by Dahua Starch Co., Ltd (Changchun, China). Eggshell powder (ESP) was made in the laboratory. Glycerol (analytical grade) was purchased from Beijing Chemical Reagent Factory (Beijing, China).
2.2. Preparation of eggshell powder (ESP)
Fresh eggshells, obtained from the waste of the school cafeteria, were washed in water to remove impurities from raw materials, and then dried in an oven at 50°C for 5 h. The dried eggshells were crushed by ultra-micro pulverizer (FW-177, Tianjin TAISITE Instrument Co., Ltd., Tianjin, China) and filtered with a 300-mesh screen. Finally, the eggshell powder dried in an oven at 50°C until constant weight was achieved. The final samples were placed in a sealed bag and stored in a desiccator.
2.3. Preparation of cornstarch composite films
The ESP with different concentrations(1, 1.5, 2, 2.5, 3 wt.%, dry starch basis) was put in a mortar and ground with a small amount of distilled water in advance, and dispersed in 30 ml distilled water and stirred by magnetic stirrer for 2 h at room temperature to make the ESP particles wet completely. Ten gram cornstarch (CS) and 5 g glycerol were dissolved in 170 ml distilled water, and the mixture was gelatinized in a water bath shaker at 90°C for 5 min. Afterward, the ESP dispersion was added dropwise to the incomplete gelatinized starch solution and the magnetic stirring was carried out for 30 min. The composite solution was heated in a water bath shaker at 90°C for 25 min to allow full gelatinization of the CS and then cooled down to room temperature. Final solution was degassed under a vacuum (0.09 MPa) for 20 min and dried on an organic glass plate (made in the laboratory, 20 × 20 cm2) for 12 h in an air-circulated oven maintaining 50°C. All the dried starch films were conditioned at 23°C and 50% relative humidity for 48 h before analysis.
2.4. Characterizations
2.4.1. Film thickness
The thickness of films was measured using digital micrometer (± 0.001 mm, Harbin, China) at eight different positions for each film sample (Chaichi et al., Citation2017; El Halal et al., Citation2017). Three replicated tests were made and the average values were used to calculate mechanical properties and water vapor permeability.
2.4.2. Particle size determination
The particle size distributions of ESP were analyzed at 25°C using a Malvern laser particle size analyzer (Mastersizer 2000, Malvern Instruments Ltd., UK).
2.4.3. Scanning electron microscopy (SEM)
The morphology characterization of ESP and films were observed using cold field emission scanning electron microscope (JSM-6700F, JEOL, Japan) with an accelerating voltage of 5 kV. Prior to the observation, all specimens were coated with a thin layer of gold under high-vacuum.
2.4.4. Fourier transform infrared spectroscopy (FTIR)
FTIR spectra for the ESP and films were measured using an FTIR spectrometer (Avatar360, Thermo Nicolet Corporation, American) through KBr module at resolution of 4 cm−1 with 16 scans. The wave numbers within the range of 500–4000 cm−1.
2.4.5. X-ray diffraction (XRD)
The eggshell powder and film samples were analyzed by X-ray diffractometer (D/Max2500, Rigaku) with high-intensity goniometer. And the instrument operated at a voltage of 40 kV and tube current of 250 mA with Cu Kα radiation. The data were collected in the range of 5◦–50◦ (2θ) with a step size of 2θ = 0.02◦, and the scanning speed was 2◦/min.
2.4.6. Mechanical properties
Mechanical properties including tensile strength (TS) and elongation at break (EB) were obtained by an auto tensile tester (XLW(B), Jinan, China) according to the ASTM D882-02 method (ASTM, Citation2002). The films were cut into 12 cm × 1.5 cm strips. The initial grip separation was 80 mm, and the cross-head speed was 200 mm/min.
2.4.7. Water vapor permeability (WVP)
The WVP of the films were determined using a moisture permeability tester (TSY-T1L, Jinan, China) based on the ASTM Standard Method E96-95 (ASTM, Citation1995) with slight modifications. The films were sealed in a permeation cup containing distilled water, and then located in the tray of weighing sensor. The test area and temperature were 63.58 cm2 and 25°C, respectively. All measurements were performed in three replicates.
2.4.8. Oxygen permeability (OP)
The OP of the films was determined using a gas permeability tester (GDP-C, Brugger, Germany) according to ASTM standard D1434-82. (ASTM, Citation2003). The samples were cut into circles (108 mm in diameter) and put between the top and bottom part of the permeation cell. Make sure that the samples were free of dust and wrinkle-free before the test. Measurements were carried out at 23°C and 0% RH. The test gas was oxygen with a purity of 99% and all measurements were performed in three replicates.
2.4.9. Thermogravimetric analysis (TGA)
Thermal degradation behavior of the pure CS film, and CS-ESP composite films were measured by thermoanalysis system (TGA-50, Shimadzu, Japan). The samples (approximately 3 mg) were heated from 40°C to 500°C at a scanning rate of 10°C/min under nitrogen atmosphere (50 ml/min). The first derivate of the weight loss curves was obtained to observe the maximum degradation temperatures of films.
2.4.10. Biodegradation test
The soil burial method was used to study the biodegradability of starch films. (Bootklad & Kaewtatip, Citation2013; Guleria, Singha, & Rana, Citation2018) The samples of films (dimensions 10 mm× 10 mm× 1.5 mm) were buried in natural soil at a depth of 7 cm in Changchun, China. The temperature was maintained at 23 ± 5°C, and the relative humidity (RH) was kept in the range of 40–50%. After 1 week, 2 weeks and 3 weeks, the samples were collected, washed with distilled water several times and dried in the oven at 60°C for 24 h. The weight loss was calculated using the following equation:
where W0 and W are the initial and final weights of the sample, respectively.
2.5. Statistical analysis
Statistical analyses of the experimental results were performed by analysis of variance (ANOVA), and Tukey’s multiple range tests were performed using a level of 95% confidence (α = 0.05).
3. Results and discussion
3.1. Characterization of ESP
Unlike universal inorganic fillers, the compositions of ESP are about 95% calcium carbonate in the form of calcite and 5% organic materials such as type X-collagen, sulfated polysaccharides, and other proteins (Lin, Zhang, & Mai, Citation2012). The organic matrix could increase the interfacial adhesion between CaCO3 particles and film-forming matrix, and improve the properties of composite films. shows the particle size distribution of ESP. The particle sizes of ESP particle at peaks Dv(10), Dv(50), and Dv(90) were obtained as 1.87 µm, 8.12 µm, and 39.7 µm, respectively. The laser particle size analyzer studies suggested that the average size of ESP is about 8.12 µm. The shape of the ESP is plate-like and jagged, as shown in and (c) (Bashir et al., Citation2017). The XRD and FTIR patterns of the ESP particles are displayed in . The characteristic diffraction peaks of ESP ()) appeared at 2θ = 23.04°, 29.4°, 31.44°, 35.98°, 39.40°, 43.16°, 47.54°, 48.52°, and the main peak at 2θ = 29.4° demonstrated that the thermodynamically stable calcite crystal is a major phase of the ESP (Rahman, Netravali, Tiimob, & Rangari, Citation2014). ) displays the FTIR patterns of ESP. It can be found that the absorption bands at 1797, 1463, 875, and 713 cm−1 are assigned to the stretching vibration of C ̶ O, asymmetric stretching vibrations of C ̶ O, out-of-plane deformation vibration of CO32-, and in-plane deformation vibration of O ̶ C ̶ O, respectively, which are the characteristic peaks of calcite. The absorption band at 2514 cm−1 may be attributed to the amine salt and the peaks at 2875 and 2977 cm−1 result from the vibration of C ̶ H, which indicated the presence of organic compounds in the ESP (Ji et al., Citation2009).
3.2. FTIR analysis
depicts the FTIR spectra of CS film and CS-ESP composite films. The absorption band occurred in CS film at approximately 707 cm−1, reflecting the typical stretching vibrations of C–C skeletons. In CS-ESP films, the absorption band (707 cm−1) shifted to a higher wave number with the addition of ESP as compared to CS film, which may be related to the changes in charge distribution caused by the induction effect from O ̶ C ̶ O bonds in the ESP. Furthermore, the skeletal mode vibration absorption bands of pyranoid ring at 574 cm−1 and 528 cm−1 for the pure CS film gradually shifted to lower wave numbers 572 cm−1 and 522 cm−1 with the increase of ESP content, respectively (Ramazan, Irudayaraj, & Seetharaman, Citation2002). This result indicated an increase in the number of hydrogen bands between starch molecules and ESP particles, which led to the vibrational frequency of the pyranoid ring skeleton decreased and the absorption bands shifted to a lower wave number. These results showed that the addition of ESP promoted the formation of hydrogen bands and reduced the number of active sites for water adsorption, which decreased the free space in the film network and strengthened the compact structure of composite films (Oleyaei et al., Citation2016). Therefore, the ability of water and oxygen resistance of the CS-ESP composite films improved.
3.3. X-ray diffraction (XRD)
The crystalline structure of starch granules consists of the ordered crystalline region and the disordered amorphous region. X-ray diffraction patterns were used to evaluate the amorphous-crystalline structure characterized by sharp peaks related to the crystalline diffraction and an amorphous zone (Li et al., Citation2015). The Wide angle X-ray diffraction patterns of the films are indicated in . The pure CS film presents an amorphous structure so that there is no obvious crystallization peak in the diffractogram, which may be related to the broke of hydrogen bond between the ordered and the disordered starch molecules because of the water molecules entered into the starch granules during the gelatinization process. Therefore, the interaction between the starch molecules was destroyed and the microcrystalline beam disintegrated.
Figure 4. The wide-angle X-ray diffraction patterns of the pure CS film and CS composite films with various ESP concentrations.
Figura 4. Patrones de difracción obtenidos por rayos-X en gran ángulo de la película del CS puro y la película de CS compuesta con varias concentraciones de la ESP.
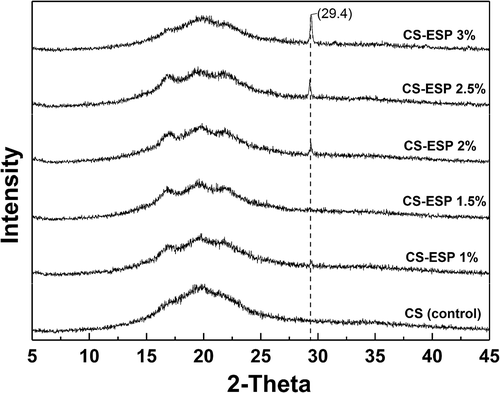
As can be seen from the diffraction patterns of the CS-ESP composite films, a new strong characteristic reflection appeared at 2θ = 29.4◦ compared to the film without the addition of ESP, which leads to a semi-crystalline structure of the composite films. The new peak was not obvious with the addition of low ESP content. This indicated that the ESP was uniformly dispersed in the CS matrix and built a strong interaction with CS (Liu, Li, & Li, Citation2017). In contrast, the intensity of diffraction peak at 2θ = 29.4◦ increased significantly as the content of ESP is greater than 2%, which may be attributed to the calcite crystal and the agglomerates of ESP. Similar observation was reported by other authors in poly(styrene-b-ethylene/butylene-b-styrene)/eggshell composites (Kang et al., Citation2010).
3.4. Scanning electron microscopy (SEM)
shows the surface and cross-section micrographs of pure CS film and CS composite films. The surface of CS film without ESP particles ()) appears a smooth (without pores and cracks) and compact structure. ()) exhibits the surface of CS-ESP2% composite films, which was observed a homogeneous and smooth structure with no cracks and obvious phase separation, and the ESP embedded in the starch matrix. This result demonstrated that the ESP particles are uniformly dispersed in the film matrix, and the ESP shows a better adhesion to the starch matrix caused by the organic components in the ESP. Consequently, the composite films have a good mechanical and barrier properties compared to control film. However, the CS-ESP3% composite films ()) shows many protuberances on the surface. This result can probably be associated with that the interface adhesion between ESP particles and CS film matrix is reduced and the structural integrity is destroyed for the composite films with high concentration of ESP. ( shows the cross-section micrographs of the films, the control film and CS-ESP2% composite films displays a continuous and smooth structure. However, the composite films with 3% ESP particles show a rough fracture with a slight protuberance. The similar structure has also been observed by other authors in the CS/CaCO3 blending films (Q. Sun, Xi, Li, & Xiong, Citation2014).
Figure 5. SEM of the surface (a1–a3) of CS, CS-ESP 2%, and CS-ESP 3% films, and the cross-section (b1–b3) of CS, CS-ESP 2%, and CS-ESP 3% films.
Figura 5. SEM de la superficie (a1–a3) de las películas de CS, CS-ESP 2% y CS-ESP 3%, y de la sección transversal (b1–b3) de las películas CS, CS-ESP 2% y CS-ESP 3%.
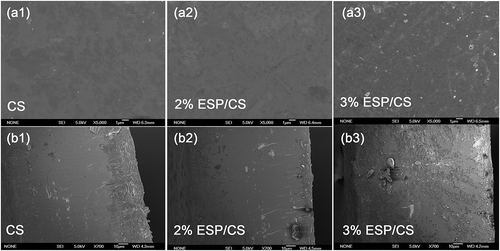
3.5. Mechanical properties
The effect of ESP on the mechanical properties of CS films plasticized with glycerol is shown in . The addition of ESP significantly improved the tensile strength (TS) and elongation at break (EB) compared to the control film. The pure CS film has the lowest TS and EB. As the concentration of ESP varied from 0% to 2%, the TS and EB of films increased from 2.37 to 4.69 MPa and 70.25–108.38%, respectively, and then they decreased with further increasing ESP content. The rise in mechanical properties might be attributed to the unique chemical compositions of ESP. On the one hand, the incorporation of CaCO3 particles in the ESP effectively enhanced the interfacial interaction between the ESP particles and CS film matrix, which reduced the mobility of the matrix chains and improved macroscopic rigidity of the CS-ESP composite films. On the other hand, there is a small amount of organic compounds on the surface of ESP, these polar groups act as coupling agents to promote the ESP uniformly dispersed in the CS matrix and improve the formation of a continuous network (Bootklad & Kaewtatip, Citation2013). The TS and EB are affected by the distribution of ESP in films and the interaction between the ESP particles and CS film matrix. As the concentration of ESP increased to 3%, the TS and EB of CS films decreased to 3.81 MPa and 98.13%, respectively. It could be attributed to the agglomeration and nonuniform dispersion of excessive ESP particles in the CS films matrix, which reduced the interaction between ESP and CS films matrix.
Table 1. The mechanical properties for CS, CS-ESP 1%, CS-ESP 1.5%, CS-ESP 2%, CS-ESP 2.5%, and CS-ESP 3% films.
Tabla 1. Propiedades mecánicas de las películas CS, CS-ESP 1%, CS-ESP 1.5%, CS-ESP 2%, CS-ESP 2.5% y CS-ESP 3%.
3.6. Barrier properties
The water vapor permeability (WVP) and oxygen permeability (OP) of films are crucial properties for food packaging materials, and they could evaluate the ability of films to make sure food safety and improve the shelf life of food products. The effect of ESP on the WVP and OP of CS films is shown in . There is a significant improvement in barrier properties with the addition of ESP. Specifically, the values of WVP and OP for the composite films are decreased from 2.17 × 10−12–1.44 × 10−12 g/cm s Pa and 2.72 × 10−5–1.22 × 10−5 cm3/m2 d Pa, respectively. It indicated that the CaCO3 promoted the formation of curved path and reduced the diffusion rate of water vapor molecules and oxygen molecules through the films. At the same time, the organic compounds on the surface of ESP increased the adhesion between CaCO3 particles and CS films matrix, which promoted the ESP dispersed the film matrix uniformly and increased the density of the CS-ESP composite films. Hence, the barrier properties of the films were improved. In addition, the enhancement in water vapor resistance is also related to the weak hydrophilicity of CaCO3 particles compared with CS, which reduced the hydrophilicity of the composite films. However, the values of WVP and OP of CS films increased with the addition of higher concentration of ESP. This might be attributed to the agglomeration of ESP particles in the CS films matrix, which destroyed the compact structure of the films.
Table 2. The WVP and OP for the pure CS film and CS composite films with various ESP concentrations.
Tabla 2. WVP y OP de las películas de CS puro y de CS compuesto con varias concentraciones de ESP.
3.7. Thermogravimetric analysis
A dynamic thermal procedure was performed from 40°C to 500°C. The thermogravimetric analysis (TGA) and derivative thermogravimetric (DTG) curves of the films are displayed in ,b). Three steps were observed from the weight loss curve of the CS films plasticized with glycerol. The initial weight loss, between 50°C and 120°C, was attributed to the evaporation of absorbed water. As the temperature increased to 150°C, the main mass loss could be associated with the decomposition of plasticizer. The last step was due to the decomposition of CS and ESP at the temperature above 250°C. The maximum degradation temperature (Tmax) and the percentage of the residues at 500°C of all the films are shown in . The Tmax and the residues percentage at 500°C for the CS film without the addition of ESP were 313.1°C and 14.05%, respectively. Compared to the control film, the CS-ESP composite films showed a higher Tmax and residual mass. These results suggested that the CS-ESP composite films have a better thermal stability than the control film and there is a good compatibility between CS and ESP.
Figure 6. TGA (a) and DTG (b) curves of CS and CS-ESP composite films with 1, 1.5, 2, 2.5, 3% (w/w) ESP.
Figura 6. Curvas TGA (a) y DTG (b) del CS y de las películas compuestas de CS-ESP con 1, 1.5, 2, 2.5, 3% (p/p) ESP.
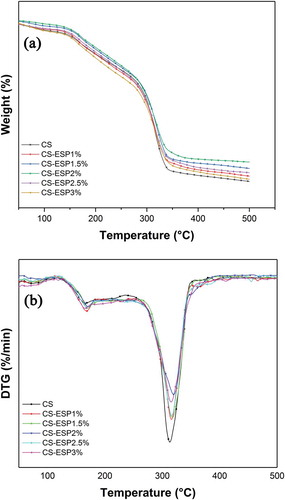
Table 3. Thermal properties for the CS and CS-ESP composite films with 1, 1.5, 2, 2.5, 3% (w/w) ESP.
Tabla 3. Propiedades térmicas del CS y de las películas compuestas CS-ESP con 1, 1.5, 2, 2.5, 3% (p/p) de ESP.
The improvement of thermal stability for composite films is attributed to the fact that the ESP affected the heat conduction of the matrix and hindered the thermal degradation of the starch films, which resulted in the Tmax hysteresis. As the addition of ESP is higher than 2%, the Tmax and the percentage of residues at 500°C are lower than the film with 2% ESP loading, but still higher than the control film. That could be attributed to the agglomeration of ESP, which decreased the thermal insulation capabilities.
3.8. Biodegradation studies
To solve environmental problems caused by non-biodegradable petroleum-based materials, biodegradable materials based on corn starch have received increasing attention because of its availability and low cost. Biodegradability of the CS film and CS-ESP2% composite film is shown in . The weight loss of CS film gradually increased with the increase of soil burial time, which proved the biodegradability of the starch film. The weight loss of CS film was 58.25% after 3 weeks of soil burial. In addition, the weight loss of the CS-ESP2% composite film was lower than the CS film. This result indicated that the incorporation of ESP promoted the formation of a continuous network and increased the density of the CS-ESP films, which reduced the effect of water and microorganisms on the composite film. This conclusion is consistent with the above research results, indicating that ESP has great potential in the field of biodegradable packaging.
4. Conclusion
In this study, a CS-ESP composite film was prepared by solution casting method. Different concentrations of ESP (1%, 1.5%, 2%, 2.5% and 3%) were incorporated into the cornstarch matrix for the reinforcement of films. We demonstrated that the incorporation of ESP increased the TS and EB simultaneously, and decreased the WVP and OP for the composite films. In addition, the ESP could improve the macroscopic rigidity and increase the interfacial adhesion between ESP particles and CS films matrix. SEM study revealed that the ESP particles are uniformly dispersed and embedded in the film matrix. FTIR analysis showed that the addition of ESP promoted the formation of hydrogen bands and strengthened the compact structure of composite films. XRD patterns indicated a semi-crystalline structure of the composite films. Analysis of thermal properties revealed that the ESP improved the thermal stability. In conclusion, the ESP as a bio-filler has great potential to improve the mechanical strength, toughness, and barrier property of films without crosslinking agent and modification in the field of food packaging.
Disclosure statement
No potential conflict of interest was reported by the authors.
Acknowledgments
This study was supported by the National Science and Technology Pillar Program of China (2015BAD16B05).
Additional information
Funding
References
- ASTM. (1995). Standard test method for water vapor transmission of materials. West Conshohocken, PA: Author. ASTM E96-95.
- ASTM. (2002). Standard test method for tensile properties of thin plastic sheeting. West Conshohocken, PA: Author. ASTM D882-02.
- ASTM. (2003). Standard test method for determining gas permeability characteristics of plastic film and sheeting. West Conshohocken, PA: Author. ASTM D1434-82.
- Bashir, A. S. M., Manusamy, Y., Chew, T. L., Ismail, H., & Ramasamy, S. (2017). Mechanical, thermal, and morphological properties of (eggshell powder)-filled natural rubber latex foam. Journal of Vinyl and Additive Technology, 23(1), 3–12.
- Bel Haaj, S., Thielemans, W., Magnin, A., & Boufi, S. (2016). Starch nanocrystals and starch nanoparticles from waxy maize as nanoreinforcement: A comparative study. Carbohydr Polym, 143, 310–317.
- Bootklad, M., & Kaewtatip, K. (2013). Biodegradation of thermoplastic starch/eggshell powder composites. Carbohydrate Polymers, 97, 315–320.
- Buakaew, W., Ruksakulpiwat, Y., Suppakarn, N., & Sutapun, W. (2013). Mechanical, thermal and morphological properties of poly(butylene succinate) filled with bio-functional filler from eggshell waste. Advanced Materials Research, 747, 72–75.
- Chaichi, M., Hashemi, M., Badii, F., & Mohammadi, A. (2017). Preparation and characterization of a novel bionanocomposite edible film based on pectin and crystalline nanocellulose. Carbohydr Polym, 157, 167–175.
- Chen, H., Shan, Z. H., Woo, M. W., & Chen, X. D. (2017). Preparation and characteristic of gelatine/oxidized corn starch and gelatin/corn starch blend microspheres. Int J Biol Macromol, 94(Pt A), 326–334.
- Chen, X., Guo, L., Du, X., Chen, P., Ji, Y., Hao, H., & Xu, X. (2017). Investigation of glycerol concentration on corn starch morphologies and gelatinization behaviours during heat treatment. Carbohydr Polym, 176, 56–64.
- Edhirej, A., Sapuan, S. M., Jawaid, M., & Zahari, N. I. (2017). Effect of various plasticizers and concentration on the physical, thermal, mechanical, and structural properties of cassava-starch-based films. Starch - Stärke, 69(1–2), 1500366.
- El Halal, S. L., Colussi, R., Biduski, B., Evangelho, J. A., Bruni, G. P., Antunes, M. D., & Zavareze, E. D. (2017). Morphological, mechanical, barrier and properties of films based on acetylated starch and cellulose from barley. J Sci Food Agric, 97(2), 411–419.
- Felix, M., Perez-Puyana, V., Romero, A., & Guerrero, A. (2017). Development of protein-based bioplastics modified with different additives. Journal of Applied Polymer Science, 134(42), 45430.
- Guleria, A., Singha, A. S., & Rana, R. K. (2018). Mechanical, thermal, morphological, and biodegradable studies of okra cellulosic fiber reinforced starch-based biocomposites. Advances in Polymer Technology, 37(1), 104–112.
- Ji, G., Zhu, H., Qi, C., & Zeng, M. (2009). Mechanism of interactions of eggshell microparticles with epoxy resins. Polymer Engineering & Science, 49(7), 1383–1388.
- Jumaidin, R., Sapuan, S. M., Jawaid, M., Ishak, M. R., & Sahari, J. (2016). Characteristics of thermoplastic sugar palm Starch/Agar blend: Thermal, tensile, and physical properties. Int J Biol Macromol, 89, 575–581.
- Kang, D. J., Pal, K., Park, S. J., Bang, D. S., & Kim, J. K. (2010). Effect of eggshell and silk fibroin on styrene–Ethylene/butylene–Styrene as bio-filler. Materials & Design, 31(4), 2216–2219.
- King’ori, A. M. (2011). A review of the uses of poultry eggshells and shell membranes. International Journal of Poultry Science, 10(11), 908–912.
- Lambert, S., & Wagner, M. (2017). Environmental performance of bio-based and biodegradable plastics: The road ahead. Chem Soc Rev, 46(22), 6855–6871.
- Li, C., Zhu, W., Xue, H., Chen, Z., Chen, Y., & Wang, X. (2015). Physical and structural properties of peanut protein isolate-gum Arabic films prepared by various glycation time. Food Hydrocolloids, 43, 322–328.
- Li, L., Chen, H., Wang, M., Lv, X., Zhao, Y., & Xia, L. (2018). Development and characterization of irradiated-corn-starch films. Carbohydr Polym, 194, 395–400.
- Lin, Z., Zhang, Z., & Mai, K. (2012). Preparation and properties of eggshell/β-polypropylene bio-composites. Journal of Applied Polymer Science, 125(1), 61–66.
- Liu, J., Liu, S., Wu, Q., Gu, Y., Kan, J., & Jin, C. (2017). Effect of protocatechuic acid incorporation on the physical, mechanical, structural and antioxidant properties of chitosan film. Food Hydrocolloids, 73, 90–100.
- Liu, S., Li, Y., & Li, L. (2017). Enhanced stability and mechanical strength of sodium alginate composite films. Carbohydr Polym, 160, 62–70.
- Liu, Z., Song, L., Zhang, F., He, W., & Linhardt, R. J. (2017). Characteristics of global organic matrix in normal and pimpled chicken eggshells. Poult Sci, 96(10), 3775–3784.
- Luchese, C. L., Spada, J. C., & Tessaro, I. C. (2017). Starch content affects physicochemical properties of corn and cassava starch-based films. Industrial Crops and Products, 109, 619–626.
- Ma, X., Cheng, Y., Qin, X., Guo, T., Deng, J., & Liu, X. (2017). Hydrophilic modification of cellulose nanocrystals improves the physicochemical properties of cassava starch-based nanocomposite films. LWT - Food Science and Technology, 86, 318–326.
- Ma, X., Lv, M., Anderson, D. P., & Chang, P. R. (2017). Natural polysaccharide composites based on modified cellulose spheres and plasticized chitosan matrix. Food Hydrocolloids, 66, 276–285.
- Malmir, S., Montero, B., Rico, M., Barral, L., Bouza, R., & Farrag, Y. (2018). Effects of poly (3-hydroxybutyrate-co-3-hydroxyvalerate) microparticles on morphological, mechanical, thermal, and barrier properties in thermoplastic potato starch films. Carbohydr Polym, 194, 357–364.
- Marcet, I., Saez, S., Rendueles, M., & Diaz, M. (2017). Edible films from residual delipidated egg yolk proteins. J Food Sci Technol, 54(12), 3969–3978.
- Miranda, C. S., Ferreira, M. S., Magalhães, M. T., Santos, W. J., Oliveira, J. C., Silva, J. B. A., & José, N. M. (2015). Mechanical, thermal and barrier properties of starch-based films plasticized with glycerol and lignin and reinforced with cellulose nanocrystals. Materials Today: Proceedings, 2(1), 63–69.
- Moreno, O., Cardenas, J., Atares, L., & Chiralt, A. (2017). Influence of starch oxidation on the functionality of starch-gelatin based active films. Carbohydr Polym, 178, 147–158.
- Murugan, S., Munusamy, Y., & Ismail, H. (2017). Effects of chicken eggshell filler size on the processing, mechanical and thermal properties of PVC matrix composite. Plastics, Rubber and Composites, 46(1), 42–51.
- Niazi, M. B. K., Jahan, Z., Berg, S. S., & Gregersen, O. W. (2017). Mechanical, thermal and swelling properties of phosphorylated nanocellulose fibrils/PVA nanocomposite membranes. Carbohydr Polym, 177, 258–268.
- Ok, Y. S., Lee, S. S., Jeon, W. T., Oh, S. E., Usman, A. R., & Moon, D. H. (2011). Application of eggshell waste for the immobilization of cadmium and lead in a contaminated soil. Environ Geochem Health, 33(Suppl 1), 31–39.
- Oleyaei, S. A., Almasi, H., Ghanbarzadeh, B., & Moayedi, A. A. (2016). Synergistic reinforcing effect of TiO2 and montmorillonite on potato starch nanocomposite films: Thermal, mechanical and barrier properties. Carbohydr Polym, 152, 253–262.
- Oleyaei, S. A., Zahedi, Y., Ghanbarzadeh, B., & Moayedi, A. A. (2016). Modification of physicochemical and thermal properties of starch films by incorporation of TiO2 nanoparticles. Int J Biol Macromol, 89, 256–264.
- Park, S., Choi, K. S., Lee, D., Kim, D., Lim, K. T., Lee, K.-H., & Kim, J. (2016). Eggshell membrane: Review and impact on engineering. Biosystems Engineering, 151, 446–463.
- Piñeros-Hernandez, D., Medina-Jaramillo, C., López-Córdoba, A., & Goyanes, S. (2017). Edible cassava starch films carrying rosemary antioxidant extracts for potential use as active food packaging. Food Hydrocolloids, 63, 488–495.
- Prabhakar, M. N., Rehaman Shah, A. U., & Song, J.-I. (2016). Fabrication and characterization of eggshell powder particles fused wheat protein isolate green composite for packaging applications. Polymer Composites, 37(11), 3280–3287.
- Quina, M. J., Soares, M. A. R., & Quinta-Ferreira, R. (2017). Applications of industrial eggshell as a valuable anthropogenic resource. Resources, Conservation and Recycling, 123, 176–186.
- Rahman, M. M., Netravali, A. N., Tiimob, B. J., & Rangari, V. K. (2014). Bioderived “Green” composite from soy protein and eggshell nanopowder. ACS Sustainable Chemistry & Engineering, 2(10), 2329–2337.
- Ramazan, K., Irudayaraj, J., & Seetharaman, K. (2002). Characterization of irradiated starches by using FT-Raman and FTIR Spectroscopy. [Journal]. J Agr Food Chem, 50(14), 3912–3918.
- Romero-Bastida, C. A., Tapia-Blacido, D. R., Mendez-Montealvo, G., Bello-Perez, L. A., Velazquez, G., & Alvarez-Ramirez, J. (2016). Effect of amylose content and nanoclay incorporation order in physicochemical properties of starch/montmorillonite composites. Carbohydr Polym, 152, 351–360.
- Saberi, B., Vuong, Q. V., Chockchaisawasdee, S., Golding, J. B., Scarlett, C. J., & Stathopoulos, C. E. (2016). Mechanical and physical properties of pea starch edible films in the presence of glycerol. Journal of Food Processing and Preservation, 40(6), 1339–1351.
- Saberi, B., Vuong, Q. V., Chockchaisawasdee, S., Golding, J. B., Scarlett, C. J., & Stathopoulos, C. E. (2017). Physical, barrier, and antioxidant properties of pea starch-guar gum biocomposite edible films by incorporation of natural plant extracts. Food and Bioprocess Technology, 10(12), 2240–2250.
- Sahari, J., Sapuan, S. M., Zainudin, E. S., & Maleque, M. A. (2013). Thermo-mechanical behaviors of thermoplastic starch derived from sugar palm tree (Arenga pinnata). Carbohydr Polym, 92(2), 1711–1716.
- Sanyang, M., Sapuan, S., Jawaid, M., Ishak, M., & Sahari, J. (2015). Effect of plasticizer type and concentration on tensile, thermal and barrier properties of biodegradable films based on sugar palm (arenga pinnata) starch. Polymers, 7(6), 1106–1124.
- Shafiur Rahman, G. M., Aftab, H., Shariful Islam, M., Mukhlish, M. Z. B., & Ali, F. (2016). Enhanced physico-mechanical properties of polyester resin film using CaCO3 filler. Fibers and Polymers, 17(1), 59–65.
- Shi, X., Jing, Z., Zhang, G., Xu, Y., & Yao, Y. (2017). Fully bio-based poly(ɛ-capolactone)/poly(lactide) alternating multiblock supramolecular polymers: Synthesis, crystallization behavior, and properties. Journal of Applied Polymer Science, 134(48), 45575.
- Song, X., Zuo, G., & Chen, F. (2018). Effect of essential oil and surfactant on the physical and antimicrobial properties of corn and wheat starch films. Int J Biol Macromol, 107(Pt A), 1302–1309.
- Souza, V. C., Niehues, E., & Quadri, M. G. N. (2016). Development and characterization of chitosan bionanocomposites containing oxidized cellulose nanocrystals. Journal of Applied Polymer Science, 133(7). doi:10.1002/app.43033.
- Sun, H., Shao, X., & Ma, Z. (2016). Effect of incorporation nanocrystalline corn straw cellulose and polyethylene glycol on properties of biodegradable films. J Food Sci, 81(10), E2529–E2537.
- Sun, Q., Xi, T., Li, Y., & Xiong, L. (2014). Characterization of corn starch films reinforced with CaCO 3 nanoparticles. PloS one, 9(9), e106727.
- Veloso Naves, M. M. F., Canuto, D., Prado, M., Marques, C., Telxeira, M., & Savio, L. (2007). Food fortification with egg shell powder as a calcium source. CIENCIA E TECNOLOGIA DE ALIMENTOS, 27(1), 99–103.
- Wang, K., Wang, W., Ye, R., Liu, A., Xiao, J., Liu, Y., & Zhao, Y. (2017). Mechanical properties and solubility in water of corn starch-collagen composite films: Effect of starch type and concentrations. Food Chem, 216, 209–216.
- Witoon, T. (2011). Characterization of calcium oxide derived from waste eggshell and its application as CO2 sorbent. Ceramics International, 37(8), 3291–3298.
- Yuan, Y., Hu, Z., Fu, X., Jiang, L., Xiao, Y., Hu, K., & Lei, J. (2016). Poly(lactic acid) plasticized by biodegradable glyceryl lactate. Journal of Applied Polymer Science, 133(21). doi:10.1002/app.43460.