ABSTRACT
The influence of heat treatment (65°C and 100°C) on the activities of potential anti-inflammatory peptides obtained from a millet protein fraction was investigated. The molecular mass of proteins was found to be in the range of 6.5–100 kDa. The protein fractions were in vitro hydrolyzed in gastrointestinal-like conditions. The highest peptide content was noted for albumin in the control sample (2.03 mg/mL). The peptide fraction obtained from globulin 7S derived from grains heated at 65°C was characterized by the highest COX-1 and COX-2 inhibitory activity (IC50 = 0.08 and 0.12 mg/mL, respectively). The peptide fraction obtained from the prolamin treatment at 100°C exhibited the highest LOX inhibitory activity (IC50 = 0.14 mg/mL). Peptides from the prolamin treatment at 65°C were characterized by the highest pancreatic lipase inhibitory activity (IC50 = 0.03 mg/mL). Peptide fractions with highest anti-inflammatory activity were rich in Gly.
Graphical abstract
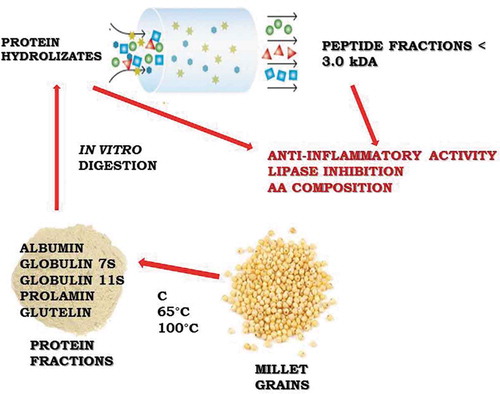
RESUMEN
Se investigó la influencia del tratamiento con calor (65°C y 100°C) en la actividad de posibles péptidos antiinflamatorios obtenidos de una fracción de proteína de mijo. Al respecto, se constató que la masa molecular de las proteínas estaba en el rango de 6.5-100 kDa. Las fracciones de proteínas se hidrolizaron in vitro en condiciones similares a las gastrointestinales. La albúmina de la muestra de control exhibió el mayor contenido de péptidos (2.03 mg/ml). La fracción peptídica obtenida de la globulina 7S derivada de granos calentados a 65°C presentó la actividad inhibidora de COX-1 y COX-2 más alta (IC50 = 0.08 y 0.12 mg/mL, respectivamente). La fracción peptídica obtenida del tratamiento con prolamina a 100°C mostró la mayor actividad inhibidora de LOX (IC50 = 0.14 mg/mL). Los péptidos del tratamiento con prolamina a 65°C exhibieron la actividad inhibidora de la lipasa pancreática más alta (IC50 = 0.03 mg/mL). Las fracciones peptídicas con mayor actividad antiinflamatoria mostraron abundante Gly.
1. Introduction
Obesity is identified as an independent and major risk factor for cardiovascular disease (CVD), chronic kidney disease, non-alcoholic fatty liver disease, type 2 diabetes, or insulin resistance (Schulte, von Eckardstein, Cullen, & Assmann, Citation2001). According to data reported by the World Health Organization (WHO) in 2016, more than 1.9 billion 18-year-old and older adults were overweight. It should be noted that over 650 million of these were obese (World Health Organization: Obesity and overweight, fact sheet, Reviewed February 2018. http://www.who.int/mediacentre/factsheets/fs311/en/). Obesity is defined as abnormal or excessive fat accumulation that may impair health. It is associated with chronic inflammation stimulating an increase in the circulating levels of pro-inflammatory cytokines such as tumor necrosis factor (TNF)-α and interleukin (IL)-6, which can induce insulin resistance and promote endothelial dysfunction (Janus, Szahidewicz-Krupska, Mazur, & Doroszko, Citation2016).
Given their anti-inflammatory, anti-pyretic, and analgesic properties, non-steroidal anti-inflammatory drugs (NSAIDs) are commonly used worldwide to treat pain and various diseases, e.g., rheumatisms or arthritis. Nevertheless, they may cause side effects such as disorders in gastrointestinal or kidney function; therefore, their use is often limited. This is the reason for considering other strategies, especially inhibition of the activity of the cyclooxygenase (COX) and lipoxygenase (LOX) enzymes, which catalyze the biosynthesis of metabolites involved in inflammatory and immune responses. Both enzymes play a key role in the catalysis of the arachidonic acid cascade, i.e. the most abundant polyunsaturated fatty acid found in cell membrane phospholipids. In this process, important bioactive fatty acids known as eicosanoids are produced (Charlier & Michaux, Citation2003). It is known that the inflammatory process plays the main role in the pathogenesis of many diseases (Co et al., Citation2018). Therefore, researchers are looking for new compounds, also food components, with a potent anti-inflammatory effect involved in inhibition of selected enzymes in the human organism. There are many studies focused on the plant protein as a source of peptides with anti-inflammatory activity (Chung, Kong, Choi, & Kong, Citation2018; González-Montoya, Hernández-Ledesma, Silván, Mora-Escobedo, & Martínez-Villaluenga, Citation2018; Kim et al., Citation2018; Montoya-Rodríguez & de Mejía, Citation2015). The anti-inflammatory mechanism of these peptides is based on the inhibition of activity of enzymes involved in the inflammatory process such as LOX, COX-1, COX-2 or inhibition of the secretion of inflammatory markers (nitric oxide, TNF-α, IL-1β, and IL-6) by macrophages. During inflammatory the process, an excess level of high inflammatory markers can lead to tissue injury, organ dysfunction, and inflammation-related tumorigenesis (Conforti & Menichini, Citation2011). Moreover, peptides with anti-inflammatory activity may inhibit inflammation-induced transforming growth factor-α (VCAM-1) and inflammatory cytokine induction by inhibiting the nuclear factor kappa-light-chain-enhancer of activated B cells (NF-κB) and the protein kinase B (AKT) signaling pathway (Burris, Ng, & Nagarajan, Citation2014).
Cyclooxygenases (COXs) are important enzymes catalyzing the biosynthesis of prostaglandin H2, which is the main precursor of production of prostaglandins, thromboxanes, and prostacyclins. There are two isoforms of COXs: cyclooxygenase-1 (COX-1) and cyclooxygenase 2 (COX-2); while both catalyze essentially the same reaction, they differ in their structure, expression, and function (Hansen et al., Citation2012). The former is a constitutive isoform mainly responsible for regulation of platelet aggregation and synthesis of prostaglandins, which have a cytoprotective effect on the gastrointestinal tract as well as renal blood flow and control renal function in the kidneys. The latter form is activated by pro-inflammatory mediators and facilitates the release of prostaglandins involved in the inflammatory process, pain, and oncogenesis (El et al., Citation2018; Hansen et al., Citation2012)
Lipoxygenases (LOXs) are a group of oxidative enzymes from the class of nonsulfur iron dioxygenases. In humans, there are six functional LOXs: 5-lipoxygenase (5-LOX), 12/15-LOX (15-LOX-1), platelet-type 12-LOX, epidermis-type (12R-LOX), epidermis-type 15-LOX (15-LOX-2), and epidermis-type ALOX3. They convert arachidonic, linoleic, and other polyunsaturated fatty acids into fatty acid hydroperoxides and biologically active metabolites such as leukotrienes (LTs) (Ribeiro et al., Citation2014; Schneider, Pratt, Porter, & Brash, Citation2009). These compounds have been reported as factors of inflammatory diseases such as atherosclerosis, rheumatoid arthritis, allergic, asthma, obesity, and certain types of cancer (Gunderson et al., Citation2016; Werz & Steinhilber, Citation2005).
On the other hand, consumption of fat-rich foods that contain triacylglycerols, i.e. the most energy-rich dietary compounds, leads first to overweight and next to obesity. One of the methods used to treat obesity is aimed at inhibition of digestion and absorption of lipids in the gastrointestinal tract followed by inhibition of production of new fat tissue. Triacylglycerols are first hydrolyzed in the stomach by acid-stable gastric lipase and next the products are transported to the duodenum, where they are substrates to the action of pancreatic lipases. This leads to release of monoglycerides and free fatty acids (FFA) (Jawed et al., Citation2018). FFAs are absorbed by enterocytes and play the main role in the synthesis of new triacylglycerols, which can be transported to various organs in the form of lipoprotein chylomicrons. Excess triglycerides from adipose tissue may lead to obesity (Mittendorfer, Citation2013), while excess free fatty acids may generate free radicals in the organism. Therefore, the inhibition of the pancreatic lipase activity may be useful in overweight and obesity treatment.
Plants have many chemical compounds with diverse structural features making many of them potentially compatible with development of an obesity-modulating mechanism. Millet is one of the gluten-free cereals and a good source of energy, protein, fatty acids, dietary fiber, polyphenols, minerals, and vitamins. It can grow in unfavorable conditions; therefore, it is often an important food in underdeveloped countries (Amadou, Gounga, & Le, Citation2013). Many health benefits of millet have been reported, e.g. blood pressure regulation or reduction of heart diseases or cholesterol levels (Gupta, Srivastava, & Pandey, Citation2012).
Moreover, millet grains are rich in protein containing a high quantity of essential amino acids, especially the sulfur-containing amino acids (methionine and cysteine), which can be a good source of bioactive peptides released during the hydrolysis process.
Cooking is one of the main and most common techniques used in food preparation. It generally reduces the nutritional quality of the product and the phytochemical content. On the other hand, this method can inactivate anti-nutritional compounds, increasing potential bioaccessibility of nutrients, or modulate the amino acid composition and protein digestibility (Margier et al., Citation2018; Nosworthy et al., Citation2018; Qi et al., Citation2019). Nowadays, it is known that the cooking conditions such as time, temperature, pH, etc. have an important influence on the nutritional value and properties of food (Becker, Boulaaba, Pingen, Krischek, & Klein, Citation2016; Kmiecik, Lisiewska, Słupski, & Gȩbczyński, Citation2008). There are many studies focused on the use of lower temperature for preparation of meat (Clausen, Christensen, Hveisel, Duelund, & Mouritsen, Citation2018) but there are no data about the impact of temperature on the activities of peptides obtained from millet grain protein fractions. It is known that parboiling as a pre-treatment stage in the preparation of millet influences the phenolic profile and in vitro digestibility of two millet products, steam-cooked couscous and porridge, made from pearl (Pennisetum glaucum) and proso (Panicum miliaceum) millets (Bora, Ragaee, & Marcone, Citation2019). Moreover, cooking in boiling water decreased the anti-nutritional factors, protein content, and in vitro protein digestibility in millet (Nour, Ahmed, Babiker, & Ibrahim, Citation2015). Generally, depending on temperature level, heat treatment causes protein denaturation and production of peptide aggregates due to formation of covalent and/or noncovalent bonds (Rombouts, Lagrain, Scherf, Koehler, & Delcour, Citation2015). Such intermolecular bonding can modulate digestibility, absorption of bioactive peptides or nutrient composition, and the color and aroma constituents of food (Qi et al., Citation2019; Wada & Lönnerdal, Citation2014). Moreover, other reactions enhanced by heat treatment of food, such as the Millard reaction, may exert a physiological effect on the bioactive peptide activity (Maestri, Pavlicevic, Montorsi, & Marmiroli, Citation2018).
The aim of this study was to investigate the influence of the heating temperature on the release of peptides from millet proteins with potential anti-inflammatory (LOX, COX-1, and COX-2 inhibition) and lipase inhibitory activity. Nowadays, low-temperature heating methods have increasingly been used in modern cooking techniques and food design. In this experiment, millet grains were cooked at 65°C or 100°C and potential anti-inflammatory and lipase inhibitory peptides obtained by heat treatment of millet grains were compared.
2. Material and methods
2.1. Material
The millet grains (Panicum miliaceum L.) were purchased from The Horticulture and Nursery Industry (PNOS) in Ożarów Mazowiecki, Poland.
2.2. Millet grains heating
The millet grains were added to distilled water at a grains/water ratio of 1:2 (w/v). The samples were heated at two temperature values of 65°C and 100°C for 30 and 15 min., respectively. Grains that were not subjected to the heating process were lyophilized and used as a control sample. All types of grains were obtained in triplicate.
2.3. Protein fractionation
The lyophilized grains were ground with a laboratory mill (MRC SM-450) and the flour was defatted with hexane at a flour/hexane ratio of 1:10 (w/v). The samples were stirred for 1 h at 4°C and next centrifuged at 8000 g for 20 min, at 4°C. The precipitate was dried in laboratory a dryer at 20°C. Defatted dry flours were kept at 4°C until used. The millet seed protein extraction was carried out according to Silva-Sánchez et al. (Citation2008). Albumin with the nonprotein nitrogen fraction was extracted from the defatted flour using distilled water (1:10, flour/water). The samples were stirred for 30 min at 4°C and centrifuged at 8000 g for 30 min. The supernatant was frozen and stored until further analysis. 7S globulin was extracted from the pellet using 0.1 M NaCl, 10 mM K2HPO4 at pH 7.5, and 1 mM EDTA. The 7S globulin samples were stirred for 30 min at 4°C and centrifuged at 8000 g for 30 min. The supernatant was frozen, stored, and used in further analysis. The resulting pellet was used for prolamin extraction using 60% aqueous ethanol. As in the case of the other proteins, the samples were stirred for 30 min at 4°C and centrifuged at 8000 g for 30 min. Glutelin was extracted from the pellet with 0.1 M NaOH. The samples were stirred for 30 min at 4°C and centrifuged at 8000 g for 30 min. All fractions were obtained in triplicate.
2.4. SDS-PAGE protein profile
The proteins from millet seeds were separated with the SDS-PAGE method using the Mini-Protean BioRad electrophoresis system (Jakubczyk, Karaś, Złotek, & Szymanowska, Citation2017). The densitograms were determined by GelAnalyzer 2010a.
2.5. Hydrolysis of millet protein fractions
All protein fractions obtained from millet seeds were subjected to in vitro hydrolysis under gastrointestinal conditions according to the method described previously (Jakubczyk et al., Citation2017). In the first step, a 4% w/v protein fraction solution was hydrolyzed with a simulated saliva solution pH = 6.75 with mM NaHCO3 and 0.35 mM NaCl. The reaction was carried out for 5 min at 37°C in darkness. The second step consisted in addition of an α-amylase solution (50 U/mg; the enzyme to substrate ratio was 1:10; w/w) and hydrolysis for 10 min at 37°C without light in an incubator (Incu-Shaker Mini, Benchmark, USA). Next, the pH of the hydrolyzates was adjusted to 2.5 using 1M HCl in order to carry out hydrolysis with pepsin (250 U/mg, the enzyme to substrate ratio was 1:100; w/w). The samples were incubated for 2 h at 37°C and next the pH value was increased to 7.0 using 1M NaOH. The last step was hydrolysis with simulated intestinal fluid (a 0.7% solution of pancreatin and a 2.5% solution of bile extract, 1:2.5, v/v) for 60 min at 37°C in the darkness. The hydrolysis was stopped by heating at 100°C for 5 min. The samples were centrifuged for 20 min at 4°C and at 8000 g for 30 min, lyophilized, and stored at −20°C.
2.6. Peptide content
In each step of the protein fraction hydrolysis, the peptide content was determined according to the method described by Adler-Nissen (Citation2002). This method is a spectrophotometric assay of the chromophore formed by the reaction of TNBS with primary amines. 95 µl of 0.1 M phosphor buffer pH = 8.2 and 50 µl 0.1% TNBS solution was added to 5 µl of the sample. The reaction was carried out at 50°C in darkness for 1 h. Next, 100 µL of 0.1 M HCl was added and, after 20 min incubation at room temperature, the absorbance at 340 nm was measured using BioTek Microplate Readers.
2.7. Fractionation of hydrolyzates
All hydrolyzates were fractionated using Amicon Ultra-15 Centrifugal Filter Units Merck Millipore (Membrane NMWL, 3kDa) and peptide fractions with molecular mass under 3.0 kDa were obtained. The fractions were lyophilized, stored at −18°C, and used in subsequent analyses.
2.8. Anti-inflammatory assay
2.8.1. COX-1 and COX-2 inhibitory assay
The effect of the peptide fraction with molecular mass under 3.0 kDa on COX-1 and COX-2 was measured using the COX Activity Assay kit from Cayman Chemical. COX activity was determined according to the instructions provided with the kit.
2.8.2. LOX inhibitory assay
The LOX inhibitory assay was carried out with the method described by Szymanowska, Jakubczyk, Baraniak, and Kur (Citation2009). A solution of pure linoleic acid was used as a substrate. 157.2 µl of pure linoleic acid was mixed with 157.2 µl of Tween-20 and 10 ml deionized water. The substrate was clarified with 1 ml of 1 M NaOH, and M/15 phosphate buffer pH = 7.0 was added to the volume of 200 ml. The enzymatic reaction mixture contained 297 µl of M/15 phosphate buffer pH = 7, 2 µl of the substrate solution, and 1 µl of the enzyme solution. A mixture of 298 µl of phosphate buffer (pH 7.0) and 2 µl of the substrate solution was used to calibrate the spectrophotometer. Changes in absorbance at 234 nm were measured using BioTek Microplate Readers.
2.9. Lipase inhibitory activity assay
Lipase inhibitory activity was determined with the method described by Jakubczyk et al. (Citation2017) with slight modification. The final volume of the reaction mixture was 150 μL (2 μL of the enzyme, 5 μL of the sample, 142 μL of 100 mM potassium phosphate buffer, pH 7.5, and 1 μL of a 100 mM p-nitrophenyl acetate (pNPA) solution in dimethyl sulfoxide (DMSO). Changes in absorbance at 234 nm were measured using BioTek Microplate Readers.
The IC50 value was determined based on assessment of the activity of all enzymes at four peptide concentrations.
2.10. Determination of the amino acid composition
The amino acid composition of the peptide fractions was determined according to the method described by White, Fry, and Hart (Citation1986). The hydrolysis of the sample (5 µg/µl) was carried out in the gas phase using 6 M HCl at 115°C for 24 h. Next, free amino acids were converted into phenylthiocarbamyl (PTC) derivatives and determined by HPLC Dionex, USA, on a PicoTag (3.9 × 150 mm) column (Waters, Milford, MA, USA). The amino acid standard was purchased from Pierce Company (USA).
2.11. Statistical analysis
All the tests were conducted in triplicate. The data are presented as means ± standard deviation (SD). Original data were used in the statistical analysis. The data were analyzed via one-way analysis of variance (ANOVA) followed by Tukey’s multiple using Statistica 13.1.
3. Results
3.1. Characteristics of the protein profile and hydrolyzates
presents SDS-PAGE protein profiles and densitometric analysis of protein bands of millet grains treated with different temperature values and the untreated control. The molecular mass of the protein was in the range from 6.5 kDa to 100 kDa. The temperature of the seed treatment had an influence on the number of protein bands; the highest number of bands was detected in the case of albumin from the non-treated control sample. Moreover, the lowest numbers of protein bands were noted for all protein fractions obtained from grains after treatment at 100°C. The molecular mass of the globulin 7S fraction was in the range from 6.5 to 70.0 kDa. Globulin 11S had been determined as a protein with molecular mass in the range from 6.5 to 70 kDa, but we observed protein bands of this fraction obtained from grains heated at 100°C with molecular mass only in two ranges from 6.5 to 20.0 kDa and 44.3 to 66.4. In turn, prolamins were characterized as low molecular mass proteins with mass under 20.0 kDa. Determination of the molecular mass of glutelin was difficult, since this fraction was not characterized by a pronounced pattern in every case. We observed a protein with molecular mass of ca. 45 kDa only in the control and samples from the grain treatment at 65°C.
Figure 1. SDS-PAGE profile and densitometric analysis of protein bands millet grains treated at 65°C (a), millet grains treated at 100°C (b), and control millet grains (c).
MW – molecular mass, A – albumin, G7 – globulin 7S, G11- globulin 11S, P – prolamin, G – glutelin.
Figura 1. Perfil de SDS-PAGE y análisis densitométrico de bandas de proteínas de granos de mijo tratados a 65 ° C (a), granos de mijo tratados a 100 ° C (b) y granos de mijo de control (c).
MW – masa molecular, A – albúmina, G7 – globulina 7S, G11 – globulina 11S, P – prolamina, G – glutelina.
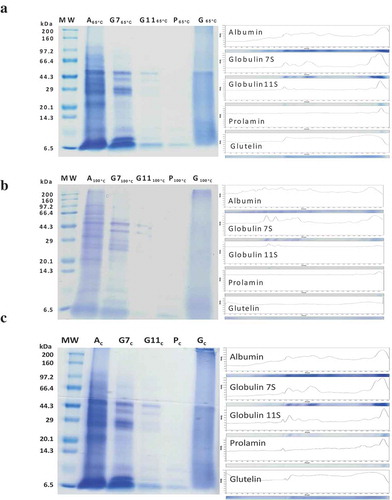
All protein fractions were hydrolyzed in vitro in gastrointestinal-like conditions. shows the peptide content after each step of hydrolysis of the protein fraction. It should be noted that the hydrolyzates obtained from the albumin fraction were characterized by the highest peptide content. Similarly, the highest peptide content was detected in the albumin fraction extracted from the control sample (2.03 mg/mL). On the other hand, the lowest peptide content was noted for globulin 11S fractions in all cases and the lowest value was exhibited by globulin 11 obtained from grains after the treatment at 65°C, where the peptide content exhibited no significant differences after hydrolysis with α-amylase and the other enzymes.
Figure 2. Peptides content of each step of hydrolysis (a – albumin hydrolysates, b – globulin 7 S hydrolysates, c – globulin 11 S hydrolysates, d – prolamin hydrolysates, e – glutelin hydrolysates).
All values are mean ± standard deviation for triplicate experiments. Different lower case letters in the same used enzyme indicate significant difference (α = 0.05). Different capital letters in the same used temperature indicate significant difference (α = 0.05).
Figura 2. Contenido de péptidos de cada etapa de hidrólisis (hidrolizados de a - albúmina, hidrolizados de b - globulina 7 S, hidrolizados de c - globulina 11 S, hidrolizados de d - prolamina, hidrolizados de e - glutelina).
Todos los valores expresan la media ± desviación estándar para experimentos por triplicado. Las diferentes letras minúsculas en la misma enzima utilizada indican una diferencia significativa (α = 0.05). Las diferentes letras mayúsculas en la misma temperatura utilizada indican una diferencia significativa (α = 0.05).
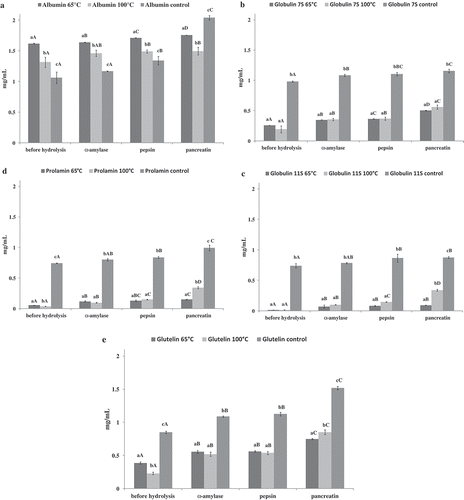
3.2. Potential anti-inflammatory activity of hydrolyzates and peptide fractions
After the hydrolysis of the protein fraction obtained from millet grains, the potential anti-inflammatory activity of the hydrolyzates was determined. presents the IC50 value of the enzyme inhibitory activity against selected enzymes involved in the inflammatory process. The lowest IC50 values were determined for all hydrolyzates obtained from the temperature treatment of grains, compared with the control sample. The highest COX-1 inhibitory activity was noted for globulin 11S and prolamin hydrolyzates obtained from grains treated with 65°C, where the IC50 values exhibited no significant differences and were 0.038 and 0.033 mg/mL, respectively. In turn, the highest COX-2 inhibitory activity was noted for hydrolysates obtained from globulin 7S treated with 100°C, where the IC50 value was 0.07 mg/mL. The highest LOX inhibitory activity was found for hydrolysates obtained from globulin 11S after the treatment at 65°C (IC50 = 0.15 mg/mL). Moreover, the lowest IC50 value of pancreatic lipase inhibitory activity, i.e. 0.37 mg/mL, was noted for glutelin treated with 65°C.
Table 1. IC50 values (mg/mL) of protein hydrolysates inhibitory activity obtained from millet grains after different temperature treatment.
Tabla 1. Valores de CI50 (mg/ml) de actividad inhibidora de hidrolizados de proteínas obtenidos de granos de mijo después de tratamientos a temperaturas diferentes.
The hydrolyzates were fractionated into the lowest peptide fractions with molecular mass under 3.0 kDa. All fractions were tested for their anti-inflammatory activity. shows the IC50 values of peptide inhibitory activity. These activities did not correspond to the hydrolyzates activity in all cases. Thus, the highest COX-1 and COX-2 inhibitory activity was noted for the peptide fraction of globulin 7S obtained from grains heated at 65°C. The IC50 values were 0.08 and 0.12 mg/mL, respectively. On the other hand, the highest LOX inhibitory activity was noted for the peptide fraction of prolamin treated with 100°C (IC50 = 0.14 mg/mL). The peptide fraction obtained also from prolamin but in the treatment at 65°C was characterized by the highest pancreatic lipase inhibitory activity (IC50 = 0.03 mg/mL).
Table 2. IC50 (mg/ml) values of peptide fractions <3.0 kDa for selected enzymes.
Tabla 2. Valores de CI50 (mg/ml) de fracciones peptídicas <3.0 kDa para enzimas seleccionadas.
3.3. Analysis of the amino acid content
The amino acid composition was determined for peptide fractions characterized by the highest anti-inflammatory activity. The peptide fraction from globulin 7S obtained in the grain treatment at 65°C (G7S 65) and prolamin yielded by the grain treatment 100°C (P 100) were selected for this analysis.
presents the percentage of content of amino acids of the peptide fractions. It should be noted that all amino acids used as a standard were detected, but the highest content was noted in the case of Gly in all fractions. In turn, the lowest content of Met was noted for G7S 65, while P 65 and P 100 were characterized by the lowest content of His.
Table 3. Amino acid composition of peptide fractions with the highest potential anti-inflammatory activity.
Tabla 3. Composición de aminoácidos de las fracciones peptídicas con mayor potencial de actividad antiinflamatoria.
4. Discussion
The molecular mass of the protein fractions was determined with the SDS-PAGE method (). The heating process had an influence on the number of protein patterns in all protein fractions. The strongest effect was observed for protein fractions obtained after the treatment at 100°C, which is associated with the process of protein denaturation (Gulati et. al, Citation2017). In this case, the protein bands in prolamin and glutelin were almost invisible. As described by Medici et al. (Citation2018), the molecular mass of the prolamin fraction was estimated at 22 kDa, while the molecular mass of glutelin extracted from two varieties of foxtail millet (white and yellow) was difficult to determine, as the SDS-PAGE image revealed many bands along the gel (Kamara, Ming, & Kexue, Citation2009). This is consistent with the results presented in this study. These differences may be due related to the differences in the protein extraction methods and plant varieties. On the other hand, after the treatment at 100°C, the most visible protein with molecular mass in the ranges of 6.5–14.14.3 and 29.0–66.4 kDa was observed in the glutelin 7S fraction. This result corresponds well to the data presented by Gulati, Sabillón, and Rose (Citation2018), where no protein patterns were noted in cooked proso millet compared with grains that were not subjected to the thermal process. Moreover, the molecular mass of proteins was similar to the value obtained in this study, where the proteins were characterized by mass in the range from 6.5 to 100 kDa, which is characteristic for this type of proteins.
All protein fractions obtained were hydrolyzed in vitro under gastrointestinal-like conditions. The enzyme used in this process is not a protease; however, as shown in our previous study (Jakubczyk et al., Citation2017), it exerts an influence on the release of peptides from proteins, which can be bound with carbohydrates. The peptide content was determined after each step of the process (). The highest peptide content was noted after the hydrolysis with pancreatic lipase for albumin hydrolyzates obtained from the control millet grains (2.03 mg/mL). The control samples exhibited the highest peptide content in all cases, except albumin, where only pancreatin digestion yielded a high value in the control sample. This may have been related to the different amino acid composition of the protein fractions (Kamara et al., Citation2009) and the fact that enzymes used during hydrolysis exhibit different protein specificities. Moreover, the temperature may have induced changes in the product and disorders in enzyme activity. It may have resulted in aggregation of denatured proteins; therefore, it was difficult to hydrolyze the protein fractions (Pan, Zeng, Bi, Claude, & Feng, Citation2016). On the other hand, the concentrations of peptides did not differ significantly in all samples, in comparison with the previous step of hydrolysis, which may have been caused by the different substrate specificity of the enzyme used or variety of protein fraction (Durak, Baraniak, Jakubczyk, & Świeca, Citation2013; Karaś, Jakubczyk, Szymanowska, Złotek, & Zielińska, Citation2017; Korhonen & Pihlanto, Citation2006).
The study results indicate that the inhibition of not only the COX activity alone but also the COX/LOX enzyme combination can be more effective and cause fewer serious side effects or limit adverse effects; therefore, such compounds can be used for design of new drugs or functional food (Rotondo, Dell, Manarini, Cerletti, & Evangelista, Citation2006).
The highest activity in this study was noted for small peptides that can get into the bloodstream; the hydrolyzates in this study were fractionated into peptides with molecular mass under 3.0 kDa. The IC50 values of enzymes involved in the pathogenesis of the inflammatory process were estimated for these peptide fractions (). It should be noted that all the values were lower than the values noted for the hydrolyzates. On the other hand, no such activity was detected in some of the hydrolyzates, especially in the control samples, which may indicate that it was induced by peptides with molecular mass above 3.0 kDa. The highest COX inhibitory activity was determined for the peptide fraction obtained from the globulin 7S hydrolyzates from millet grains heated at 65°C. The IC50 value was 0.08 mg/mL for COX-1 and 0.12 mg/mL for COX-2. Noteworthy, compounds or drugs that inhibit not only COX-1 activity but also COX-2 activity may limit adverse effects (Cuniberti et al., Citation2012). Endogenous peptides have been identified as anti-inflammatory factors and it is known that they can be used in therapies of inflammatory or autoimmune diseases (Gonzalez-Rey, Anderson, & Delgado, Citation2007). Moreover, anti-inflammatory activity via inhibition of the signal transduction pathways for expression of inflammatory cytokines of several synthetic and natural peptides has been described. A study on mice with neuropathological symptoms of Alzheimer’s disease has shown that intranasal treatment with amyloid-beta (Abeta) peptide significantly decreased A-beta plaque burden along with anti-inflammatory cytokines such as IL-4 and IL-10 (Weiner et al., Citation2000). Similarly, several synthetic peptides cause inhibition of the inflammatory process by inactivation of early signal transduction pathways of inflammatory cytokines (Gonzalez et al., Citation2005). The elucidation of the correlation between the amino acid composition in peptides and their anti-inflammatory properties may contribute to designing peptides with high in vivo anti-inflammatory activity. A study on the composition of peptides inhibiting the inflammatory process has indicated that the main role is played by the dominance of leucine, serine, tyrosine, and arginine residues, compared to non anti-inflammatory epitopes. Moreover, the hydrophobic and polar residues of peptides were determined as specific motifs of anti-inflammatory epitopes (Gupta, Sharma, Shastri, Madhu, & Sharma, Citation2017).
The peptide fractions obtained from the prolamin hydrolyzates from millet grains treated with 65°C and 100°C were characterized by the highest LOX and lipase inhibitory activity, respectively. Lipase inhibitory activity was detected only in peptide fractions from grains subjected to the temperature treatment, while no such activity was detected in the control sample. This may suggest that the thermal or another process such as fermentation modifies the properties of peptides obtained from proteins (Jakubczyk et al., Citation2017; Karaś et al., Citation2015).
The amino acid composition was investigated in the peptide fraction with the highest anti-inflammatory activity (). All amino acids used as a standard were detected in the samples, with the highest content of Gly. It should be noted that glycine-rich proteins have been characterized as novel stress-response proteins produced by plants during growth in different conditions. These proteins are found in a large number in plants and play the main role in the stress tolerance mechanism or genetic improvement through production of a plant variety with higher stress tolerance (Czolpinska & Rurek, Citation2018). Moreover, glycine was characterized as a new anti-inflammatory immunonutrient that may be useful in the treatment of many neurodegenerative diseases, especially as an inhibitory neurotransmitter, or inflammatory diseases such as sepsis, arthritis, or adult respiratory distress syndrome (Wheeler et al., Citation1999). This amino acid residue has also been described as an inhibitor of NF-κB activation, IκBα degradation, CD62E expression, and IL-6 production in HCAECs, suggesting that it may exert anti-inflammatory effects during endothelial inflammation (Hasegawa et al., Citation2012).
There are a few studies of peptides with potent anti-inflammatory activity but the exact mechanism of inhibition of enzymes involved in the pathogenesis of the inflammatory process is still unclear. The results of the study described by Montoya-Rodríguez and de Mejía (Citation2015) indicated that pure amaranth peptides HGSEPFGPR and RPRYPWRYT reduced the expression of LOX-1 and other factors involved in the inflammatory process such as lipopolysaccharide (LPS)-induced inflammation or transforming growth factor-α(VCAM-1)-induced inflammation.
The inhibition of lipase activity can be used for determination of the potential suitability of food products or food compounds to serve as anti-obesity agents (de la Garza, Milagro, Boque, Campión, & Martínez, Citation2011). On the other hand, this activity can indirectly influence the inhibition of inflammatory process, since obesity is associated with chronic inflammation and is a major risk factor for many diseases such as diabetes, chronic kidney disease, and cardiovascular diseases (Toita, Kawano, Murata, & Kang, Citation2016). The lipolytic mechanism of lipases and their role in the pathogenesis of inflammation and obesity is still poorly understood. Orlistat is one of the well-known anti-obesity agents inducing inhibition of lipase activity by interaction with the catalytic sites of the enzyme. Two peptides obtained from soybean with sequences EITPEKNPQLR and RKQEEDEDEEQQRE and described by (Martinez-Villaluenga, Rupasinghe, Schuler, & De, Citation2010) exhibited a similar manner of blocking the catalytic domain of the enzyme. Peptides with sequences INEGSLLLPH, FVVAEQAGNEEGFE, SGGGGGGVAGAATASR, GSGGGGGGGFGGPRR, INEGSLLLPH, GGYQGGGYGGNSGGGYGNRG, GGSGGGGGSSSGRRP, and GDTVTVEFDTFLSR obtained from fermented bean exhibited lipase inhibitory activity (Jakubczyk et al., Citation2017).
In this study, all protein amino acids were detected in potentially bioavailable but non-purified peptide fractions; this indicates that the correlation between the amino acid composition in the peptide fraction should still be investigated (Gupta et al., Citation2017).
5. Conclusion
Millet grains are the most common cereal used for food preparation in many cultures worldwide. It is known that the cooking technique has an influence on the activities and properties of food. In this study, the influence of different temperature values (65°C and 100°C) on the anti-inflammatory activity of peptides obtained from different protein fractions was investigated. It should be noted that the process temperature had an effect on the number of protein patterns, especially in samples treated with 100°C. The highest peptide content was noted for the control sample, which indicated that temperature induced changes in the product. The inhibitory activity of the protein hydrolyzates was lower than the activity of the peptide fraction with molecular mass under 3.0 kDa. It should be noted that not all activities were detected; this indicates that some of them were caused by peptides with higher molecular mass. The peptides with higher anti-inflammatory activity exhibited the highest content of Gly. In conclusion, the temperature of the millet preparation process had an influence on the anti-inflammatory activity of peptides obtained from millet protein fractions. Moreover, the mechanism of the anti-inflammatory activity of these peptides should be investigated more profoundly.
Acknowledgments
The scientific work was financed by Ministry of Science and Higher Education from the budget for science in the years 2016-2019 project no IP2015 026174 “Iuventus Plus V”.
Disclosure statement
No potential conflict of interest was reported by the authors.
Additional information
Funding
References
- Adler-Nissen, J. (2002). Determination of the degree of hydrolysis of food protein hydrolysates by trinitrobenzenesulfonic acid. Journal of Agricultural and Food Chemistry, 27, 1256–1262.
- Amadou, I., Gounga, M. E., & Le, G. W. (2013). Millets: Nutritional composition, some health benefits and processing – A review. Emirates Journal of Food and Agriculture, 25(7), 501–508.
- Becker, A., Boulaaba, A., Pingen, S., Krischek, C., & Klein, G. (2016). Low temperature cooking of pork meat — Physicochemical and sensory aspects. Meat Science, 118, 82–88.
- Bora, P., Ragaee, S., & Marcone, M. (2019). Effect of parboiling on decortication yield of millet grains and phenolic acids and in vitro digestibility of selected millet products. Food Chemistry, 274, 718–725.
- Burris, R. L., Ng, H. P., & Nagarajan, S. (2014). Soy protein inhibits inflammation-induced VCAM-1 and inflammatory cytokine induction by inhibiting the NF-κB and AKT signaling pathway in apolipoprotein E-deficient mice. European Journal of Nutrition, 53(1), 135–148.
- Charlier, C., & Michaux, C. (2003). Dual inhibition of cyclooxygenase-2 (COX-2) and 5-lipoxygenase (5-LOX) as a new strategy to provide safer non-steroidal anti-inflammatory drugs. European Journal of Medicinal Chemistry, 38(7–8), 645–659.
- Chung, J. H., Kong, J. N., Choi, H. E., & Kong, K. H. (2018). Antioxidant, anti-inflammatory, and anti-allergic activities of the sweet-tasting protein brazzein. Food Chemistry, 267, 163–169.
- Clausen, M. P., Christensen, M., Hveisel, T., Duelund, L., & Mouritsen, O. G. (2018). The quest for umami: Can sous vide contribute? International Journal of Gastronomy and Food Science, 13, 129–133.
- Co, F., Bri, A., Mestres, C., Alter, P., Durand, N., & Bohuon, P. (2018). Multi-response modeling of reaction-diffusion to explain alpha-galactosidase behavior during the soaking-cooking process in cowpea. Food Chemistry, 242, 279–287.
- Conforti, F., & Menichini, F. (2011). Foods of plant origin as source of nitric oxide production inhibitors. Handbook of nutritional biochemistry: genomics, metabolomics, and food supply. Nova Science Publishers, Inc.
- Cuniberti, B., Odore, R., Barbero, R., Cagnardi, P., Badino, P., Girardi, C., & Re, G. (2012). In vitro and ex vivo pharmacodynamics of selected non-steroidal anti-inflammatory drugs in equine whole blood. Veterinary Journal, 191, 327–333.
- Czolpinska, M., & Rurek, M. (2018). Plant glycine-rich proteins in stress response: An emerging, still prospective story. Frontiers in Plant Science, 9, 1–13.
- de la Garza, A. L., Milagro, F. I., Boque, N., Campión, J., & Martínez, J. A. (2011). Natural inhibitors of pancreatic lipase as new players in obesity treatment. Planta Medica, 77(8), 773–785.
- Durak, A., Baraniak, B., Jakubczyk, A., & Świeca, M. (2013). Biologically active peptides obtained by enzymatic hydrolysis of Adzuki bean seeds. Food Chemistry, 141(3), 2177–2183.
- El, M. T., El-Sharief, M. A. M. S., Zarie, E. S., Morsy, N. M., Elsheakh, A. R., Voronkov, A., … Hassan, G. S. (2018). Design, synthesis, anti-inflammatory activity and molecular docking of potential novel antipyrine and pyrazolone analogs as cyclooxygenase enzyme (COX) inhibitors. Bioorganic & Medicinal Chemistry Letters, 28(5), 952–957.
- Gonzalez, R. R., Fong, T., Belmar, N., Saban, M., Felsen, D., & Te, A. (2005). Modulating bladder neuro-inflammation: RDP58, a novel anti-inflammatory peptide, decreases inflammation and nerve growth factor production in experimental cystitis. Journal of Urology, 173(2), 630–634.
- González-Montoya, M., Hernández-Ledesma, B., Silván, J. M., Mora-Escobedo, R., & Martínez-Villaluenga, C. (2018). Peptides derived from in vitro gastrointestinal digestion of germinated soybean proteins inhibit human colon cancer cells proliferation and inflammation. Food Chemistry, 242, 75–82.
- Gonzalez-Rey, E., Anderson, P., & Delgado, M. (2007). Emerging roles of vasoactive intestinal peptide: A new approach for autoimmune therapy. Annals of the Rheumatic Diseases, 66, 70–76.
- Gulati, P., Li, A., Holding, D., Santra, D., Zhang, Y., & Rose, D. (2017). Heating reduces proso millet protein digestibility via formation of hydrophobic aggregates. Journal Of Agricultural and Food Chemistry, 65, 1952–1959. doi: 10.1021/acs.jafc.6b05574.
- Gulati, P., Sabillón, L., & Rose, D. J. (2018). Effects of processing method and solute interactions on pepsin digestibility of cooked proso millet flour. Food Research International, 109, 583–588.
- Gunderson, C. C., Ding, K., Dvorak, J., Moore, K. N., McMeekin, D. S., & Benbrook, D. M. (2016). The pro-inflammatory effect of obesity on high grade serous ovarian cancer. Gynecologic Oncology, 143(1), 40–45.
- Gupta, N., Srivastava, A. K., & Pandey, V. N. (2012). Biodiversity and nutraceutical quality of some Indian millets. Proceedings of the National Academy of Sciences India Section B - Biological Sciences, 82(2), 265–273.
- Gupta, S., Sharma, A. K., Shastri, V., Madhu, M. K., & Sharma, V. K. (2017). Prediction of anti-inflammatory proteins/peptides: An insilico approach. Journal of Translational Medicine, 15, 1–11.
- Hansen, F. K., Khankischpur, M., Tolaymat, I., Mesaros, R., Dannhardt, G., & Geffken, D. (2012). Efficient synthesis and 5-LOX/COX-inhibitory activity of some 3-hydroxybenzo-β-thiophene-2-carboxylic acid derivatives. Bioorganic and Medicinal Chemistry Letters, 22(15), 5031–5034.
- Hasegawa, S., Ichiyama, T., Sonaka, I., Ohsaki, A., Okada, S., Wakiguchi, H., … Furukawa, S. (2012). Cysteine, histidine and glycine exhibit anti-inflammatory effects in human coronary arterial endothelial cells. Clinical and Experimental Immunology, 167(2), 269–274.
- Jakubczyk, A., Karaś, M., Złotek, U., & Szymanowska, U. (2017). Identification of potential inhibitory peptides of enzymes involved in the metabolic syndrome obtained by simulated gastrointestinal digestion of fermented bean (Phaseolus vulgaris L.) seeds. Food Research International, 100, 489–496.
- Janus, A., Szahidewicz-Krupska, E., Mazur, G., & Doroszko, A. (2016). Insulin resistance and endothelial dysfunction constitute a common therapeutic target in cardiometabolic disorders. Mediators of Inflammation, 2016, 10. Article ID 3634948
- Jawed, A., Singh, G., Kohli, S., Sumera, A., Haque, S., Prasad, R., & Paul, D. (2018). Therapeutic role of lipases and lipase inhibitors derived from natural resources for remedies against metabolic disorders and lifestyle diseases. South African Journal of Botany, 120, 25–32.
- Kamara, M. T., Ming, Z. H., & Kexue, Z. (2009). Extraction, characterization and nutritional properties of two varieties of defatted foxtail millet flour (Setaria italica L.) grown in China. Asian Journal of Biochemistry,4, 88–98. doi:10.3923/ajb.2009.88.98
- Karaś, M., Baraniak, B., Rybczyńska, K., Gmiński, J., Gaweł-Bęben, K., & Jakubczyk, A. (2015). The influence of heat treatment of chickpea seeds on antioxidant and fibroblast growth-stimulating activity of peptide fractions obtained from proteins digested under simulated gastrointestinal conditions. International Journal of Food Science and Technology, 50(9), 2097–2103.
- Karaś, M., Jakubczyk, A., Szymanowska, U., Złotek, U., & Zielińska, E. (2017). Digestion and bioavailability of bioactive phytochemicals. International Journal of Food Science and Technology, 52(2), 291–305.
- Kim, M. Y., Jang, G. Y., Lee, Y. J., Woo, K. S., Hwang, B. Y., Lee, J., & Jeong, H. S. (2018). Identification of anti-inflammatory active peptide from black soybean treated by high hydrostatic pressure after germination. Phytochemistry Letters, 27, 167–173.
- Kmiecik, W., Lisiewska, Z., Słupski, J., & Gȩbczyński, P. (2008). Effect of preliminary and culinary processing on amino acid content and protein quality in frozen French beans. International Journal of Food Science and Technology, 43(10), 1786–1791.
- Korhonen, H., & Pihlanto, A. (2006). Bioactive peptides: Production and functionality. International Dairy Journal, 16, 945–960.
- Maestri, E., Pavlicevic, M., Montorsi, M., & Marmiroli, N. (2018). Meta-analysis for correlating structure of bioactive peptides in foods of animal origin with regard to effect and stability. Comprehensive Reviews in Food Science and Food Safety, 18, 3–30.
- Margier, M., Georgé, S., Hafnaoui, N., Remond, D., Nowicki, M., Du Chaffaut, L., … Reboul, E. (2018). Nutritional composition and bioactive content of legumes: Characterization of pulses frequently consumed in France and effect of the cooking method. Nutrients, 10(11), 1–12.
- Martinez-Villaluenga, C., Rupasinghe, S. G., Schuler, M. A., & De, E. G. (2010). Peptides from purified soybean β-conglycinin inhibit fatty acid synthase by interaction with the thioesterase catalytic domain. Food Chemistry, 277, 1481–1493.
- Medici, L. O., Gonçalves, F. V., Da Fonseca, M. P. S., Gaziola, S. A., Schmidt, D., Azevedo, R. A., & Pimentel, C. (2018). Growth, yield and grain nutritional quality in three Brazilian pearl millets (Pennisetum americanum L.) with African or Indian origins. Anais Da Academia Brasileira de Ciencias, 90(2), 1749–1758.
- Mittendorfer, B. (2013). Origins of metabolic complications in obesity: Adipose tissue and free fatty acid trafficking. Current Opinion in Clinical Nutrition and Metabolic Care, 14(6), 535–541.
- Montoya-Rodríguez, A., & de Mejía, E. G. (2015). Pure peptides from amaranth (Amaranthus hypochondriacus) proteins inhibit LOX-1 receptor and cellular markers associated with atherosclerosis development in vitro. Food Research International, 77, 204–214.
- Nosworthy, M. G., Medina, G., Franczyk, A. J., Neufeld, J., Appah, P., Utioh, A., … House, J. D. (2018). Effect of processing on the in vitro and in vivo protein quality of beans (Phaseolus vulgaris and Vicia Faba). Nutrients, 10(6), 1–13.
- Nour, A. A. M., Ahmed, I. A. M., Babiker, E. E., & Ibrahim, M. A. E. M. (2015). Effect of supplementation and cooking on in vitro protein digestibility and anti-nutrients of pearl millet flour. American Journal of Food Science and Health, 1(3), 69–75.
- Pan, A., Zeng, H., Bi, G., Claude, F., & Feng, B. (2016). Heat-pretreatment and enzymolysis behavior of the lotus seed protein. Food Chemistry, 201, 230–236.
- Qi, X., Cheng, L., Li, X., Zhang, D., Wu, G., Zhang, H., … Wang, Y. (2019). Effect of cooking methods on solubility and nutrition quality of brown rice powder. Food Chemistry, 274, 444–451.
- Ribeiro, D., Freitas, M., Tomé, S. M., Silva, A. M. S., Porto, G., Cabrita, E. J., … Fernandes, E. (2014). Inhibition of LOX by flavonoids: A structure-activity relationship study. European Journal of Medicinal Chemistry, 72, 137–145.
- Rombouts, I., Lagrain, B., Scherf, K. A., Koehler, P., & Delcour, J. A. (2015). Formation and reshuffling of disulfide bonds in bovine serum albumin demonstrated using tandem mass spectrometry with collision-induced and electron-transfer dissociation. Scientific Reports, 5, 1–12.
- Rotondo, S., Dell, G., Manarini, S., Cerletti, C., & Evangelista, V. (2006). The lipoxygenase – Cyclooxygenase inhibitor licofelone prevents thromboxane A2-mediated cardiovascular derangement triggered by the inflammatory peptide fMLP in the rabbit. European Journal of Pharmacology, 546, 95–101.
- Schneider, C., Pratt, D. A., Porter, N. A., & Brash, A. R. (2009). Control of oxygenation in lipoxygenase and cyclooxygenase catalysis. Chemistry & Biology, 14(5), 473–488.
- Schulte, H., von Eckardstein, A., Cullen, P., & Assmann, G. (2001). Obesity and cardiovascular risk. Herz, 26(3), 170–177.
- Silva-Sánchez, C., de la Rosa, A. P. B., León-Galván, M. F., de Lumen, B. O., de León-Rodríguez, A., & de Mejía, E. G. (2008). Bioactive peptides in amaranth seed. Journal of Agricultural and Food Chemistry, 56(4), 1233–1240.
- Szymanowska, U., Jakubczyk, A., Baraniak, B., & Kur, A. (2009). Characterisation of lipoxygenase from pea seeds (Pisum sativum var. Telephone L.). Food Chemistry, 116(4), 906–910.
- Toita, R., Kawano, T., Murata, M., & Kang, J. (2016). Biomaterials anti-obesity and anti-inflammatory effects of macrophage-targeted interleukin-10-conjugated liposomes in obese mice. Biomaterials, 110. 81–88. doi: 10.1016/j.biomaterials.2016.09.018
- Wada, Y., & Lönnerdal, B. (2014). Bioactive peptides derived from human milk proteins – Mechanisms of action. Journal of Nutritional Biochemistry, 25(5), 503–514.
- Weiner, H. L., Lemere, C. A., Maron, R., Spooner, E. T., Grenfell, T. J., Mori, C., & Issazadeh, S. (2000). Nasal Administration of amyloid-β peptide decreases cerebral amyloid burden in a mouse model of Alzheimer’s Disease. Annals of Neurology, 48, 567–579.
- Werz, O., & Steinhilber, D. (2005). Development of 5-lipoxygenase inhibitors – Lessons from cellular enzyme regulation. Biochemical Pharmacology, 70(3), 327–333.
- Wheeler, M. D., Ikejema, K., Enomoto, N., Stacklewitz, R. F., Seabra, V., Zhong, Z., … Thurman, R. G. (1999). Glycine: A new anti-inflammatory immunonutrient. Cellular and Molecular Life Sciences, 56(9–10), 843–856.
- White, J. A., Fry, J. C., & Hart, R. J. (1986). An evaluation of the Waters Pico Tag System for the amino acid analysis of food materials. Journal of Automatic Chemistry, 8(4), 170–177.