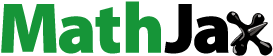
ABSTRACT
This study assessed the gross-structure, micro-structure, physical characteristics and nutritional composition of Trichilia emetica and Trichilia dregeana seeds. T. emetica and T. dregeana seeds have potential for commercialization and improved food security, yet they are under-utilized and under-researched. The gross- and micro-structure of the seeds was assessed using stereo microscopy, light microscopy (LM), confocal laser scanning microscopy (CLSM) and scanning electron microscopy (SEM). Protein, fat, ash, and mineral content of the seeds were also assessed. T. emetica and T. dregeana seeds had lower bulk density (393.5 kgm−3 & 433.6 kgm−3 respectively) and lower porosity (55.07% & 54.38% respectively) than soybean. Geometric mean diameter (29.7 mm & 16.9 mm) and aspect ratio (1.72 and 1.85) of T. emetica and T. dregeana seeds respectively, were higher than soybean, while they had lower sphericity (0.66 and 0.66 respectively) than soybean (0.99). Microscopy analysis showed that Trichilia species had larger-sized and more round-shaped protein bodies than soybean seeds. T. emetica and T. dregeana protein contents (25.6% and 17.3% w/w, respectively) were lower than soybean (45.4%) while fat contents (49% and 51.5% w/w, respectively) were higher than soybean (20.2% w/w). Potassium (1075–1350 mg100g−1) and calcium (285–300 mg100g−1) where the main macro-minerals while iron (6.33–6.83 mg100g−1) and zinc (2.30–2.90 mg100g−1) were the main micro-minerals. The research demonstrated that the structural and nutritional characteristics of T. dregeana and T. emetica seeds could facilitate their commercial utilization and application for food security alleviation.
RESUMEN
Este estudio evaluó la estructura bruta, la microestructura, las características físicas y la composición nutricional de las semillas de Trichilia emetica y Trichilia dregeana. Si bien las semillas de T. emetica y T. dregeana poseen potencial para ser comercializadas y mejorar la seguridad alimentaria, son infrautilizadas y han sido poco investigadas. Para evaluar algunos de los aspectos mencionados —estructura bruta y microestructura— de las semillas se emplearon microscopía estereoscópica, microscopía de luz (LM), microscopía confocal de barrido láser (CLSM) y microscopía electrónica de barrido (SEM). Además, se evaluó el contenido de proteínas, grasas, cenizas y minerales de las mismas. Se constató que las semillas de T. emetica y T. dregeana tienen una densidad aparente menor (393.5 kgm−3 y 433.6 kgm−3, respectivamente) y una porosidad menor (55.07% y 54.38%, respectivamente) que las de la soya. El diámetro geométrico medio (29.7 mm y 16.9 mm) y la ratio de aspecto (1.72 y 1.85) de las semillas de T. emetica y T. dregeana, respectivamente, son mayores que los de la soya, mientras que su esfericidad es menor (0.66 y 0.66, respectivamente) que la de la soya (0.99). El análisis microscópico permitió comprobar que las especies de Trichilia poseen cuerpos proteicos de mayor tamaño que las semillas de soya y que su forma es redonda. Asimismo, se constató que los contenidos proteicos de T. emetica y T. dregeana (25.6% y 17.3% p/p, respectivamente) son inferiores a los de la soya (45.4%), mientras que los contenidos grasos (49% y 51.5% p/p, respectivamente) superan los de la soya (20.2% p/p). Los principales macrominerales detectados son potasio (1075-1350 mg100g−1) y calcio (285-300 mg100g−1), y los principales microminerales hierro (6.33-6.83 mg100g−1) y zinc (2.30-2.90 mg100g−1). La investigación dio cuenta de que las características estructurales y nutricionales de las semillas de T. dregeana y T. emetica podrían facilitar su utilización comercial y su aplicación para garantizar la seguridad alimentaria.
1. Introduction
Food insecurity is an on-going challenge in various parts of the world especially at household level. Globally, food insecurity affects more than a quarter (26.4%) of the world population and is associated with malnutrition and under-nutrition (Cafiero et al., Citation2018; Huizar et al., Citation2020). The T. emetica and T. dregeana seeds represent an underutilized and under-researched potential valuable food source that may improve food and nutrition security which could become comparable to sunflower, soybean, canola and peanut. Commercial utilization of Trichilia species seeds may lead to improved food and nutrition security. The physical difference between the two Trichilia species is that T. dregeana fruits grow directly from the leafstalk whereas the T. emetica fruits are attached to the fruit stem with a distinct stipe (South African National Biodiversity Institute, Citation2004). There are 260 species of Trichilia (Usman et al., Citation2018), commonly found in African countries while T. emetica and T. dregeana are also widely distributed throughout America. Utilization of the Trichilia spp remains domestic and in African rural areas T. emetica is used as a complementary food to alleviate malnutrition (Komane et al., Citation2011; Van Wyk et al., Citation2000). The seeds can be soaked in water to produce a milky juice which is then mixed with vegetables such as spinach or sweet-potatoes or squash to make delicious dishes (Komane et al., Citation2011; South African National Biodiversity Institute, Citation2004). Trichilia oil is edible and used to preserve food products as well as cooking in rural communities (Komane et al., Citation2011). Traditionally, Trichilia oil was used in the production of natural soaps, candle making, hair oil, body ointment and lip balm (Komane et al., Citation2011). Trichilia spp non-seed parts are important in Africa for their traditional medicine purposes such as to treat leprosy, skin ailments, kidney problems, insomnia, stomach complaints, pneumonia, colds and backaches (South African National Biodiversity Institute, Citation2004; Vermaark et al., Citation2011). Trichilia plants are wild, evergreen plants and are available throughout the year (South African National Biodiversity Institute, Citation2004).
Knowledge of seed gross structure, microstructure, physical and nutritional properties may be vital for utilization and commercialization of the seeds as an alternative food source (Aguilera, Citation2005; Prada & Aguilera, Citation2007). The physical properties include hardness, geometric mean diameter, shape, size, surface area and density. Knowledge of these properties can facilitate the design of equipment for harvesting, handling, cleaning, sorting, dehulling, packaging, storage, transport, and milling operations (Niveditha et al., Citation2013; Sadowska et al., Citation2013; Wandkar et al., Citation2012). Seed microstructure is also important in understanding the food material functionality of seeds (Aguilera, Citation2005; Prada & Aguilera, Citation2007).
To the best of our knowledge, there is no information on seed gross structure, microstructure and physical properties for T. emetica and T. dregeana seeds. In addition, information on the nutritional composition of Trichilia spp (emetica and dregeana) is very limited and hence requires further investigation. Therefore, the present study investigated the gross structure, microstructure, physical characteristics, and nutritional properties (composition) of T. emetica and T. dregeana seeds. The results contribute to the utilization of the seeds for commercial and food security purposes.
2. Materials and methods
2.1. Materials
Trichilia seeds (T. emetica, T. dregeana) and soybean (Glycine max (L.) Merrill) seeds were used. The T. emetica and T. dregeana seeds were harvested (May 2017 to August 2018) at the Agricultural Research Council-Tropical and Subtropical Crops (ARC-TSC), Mbombela (−25.45127 S 30.96919 E), South Africa. The soybean seeds, which served as a reference oilseed, were obtained from the Agricultural Research Council-Grain Crops (ARC-GC) campus in Potchefstroom (26.72866 S 27.07972 E), South Africa. The T. emetica and T. dregeana seeds were distinguished from each other based on their leaves, fruit-stem attachment and fruit morphology (Van Wyk et al., Citation2000). Trichilia spp trees used were more than 10 years old and the seeds were selected according to fruit-stem attachment and seed size. Trichilia trees were cultivated at ARC-TSC (Mbombela, South Africa) for agronomic assessment, ornamental purposes, and shade. The T. emetica, T. dregeana and soybean seeds were dried in an oven (AB 3000 Agridrier, Dryer for Africa, South Africa) at 50°C with a RH of 15% for 16 h. The seeds were then freeze dried (Vir Tris Bench Top Pro with Omnitronics, SP Scientific, St Louis, Pennsylvania) at −40°C with 65 Pa for 4 days.
2.2. Methods
2.2.1. Physical and functional properties of the seeds
The average unit volume (V1), bulk density (ρb) and porosity of T. emetica, T. dregeana and soybean seeds were determined by the fluid displacement method described by Pereas-Flores et al. (Citation2011). A 500 mL graduated cylinder was filled with sunflower oil (190 mL) and 30 seeds were added. The average unit volume (V1) was determined by applying the following formula:
Bulk density (ρb) was calculated as the ratio of mass to volume that was filled with the seeds (n = 30). The true density (was determined by the benzene displacement method. A 50 mL graduated cylinder was filled with toluene (benzene) (20 mL) and seeds were added. The true density was calculated as the ratio of the mass of the sample to its true volume. Bulk and true densities were expressed as kgm−3. The porosity (
) of T. emetica, T. dregeana and soybean seeds were expressed in % and were calculated using the following formula:
The geometric mean diameter (GMD) (Dg) was calculated as the relationship between the length, width and thickness of the seed. The length (L), width (W) and thickness (T) of T. emetica, T. dregeana and soybean seeds were measured using a stereo microscope. A total of 60 seeds were selected by quartering, and on each individual seed the length, width and thickness were determined. The stereo microscopic analysis for dried T. emetica, T. dregeana and soybean whole seeds were done according to Mohsenin (Citation1970) and Pereas-Flores et al. (Citation2011). An analytical sample (n > 30 seeds) was obtained by the quartering method. The seeds were examined using a Zeiss Stereo Discovery. V20 microscope (Carl Zeiss, Germany) at 20X magnification. Geometric mean diameter was expressed in mm.
The Aspect Ratio (AR) is a relationship between the minimum and maximum diameters. The value 1 for AR is related to equi-dimensional objects and tends to infinity for extended objects (Pereas-Flores et al., Citation2011). AR was determined using the following formula:
The sphericity () of T. emetica, T. dregeana and soybean seeds were calculated according to Mohsenin (Citation1970) and Pereas-Flores et al. (Citation2011) using the following formula:
2.2.2. Macro-nutrient composition
The macro-nutrient composition is a routine analysis that is used to provide classification of the seeds components. This analysis is composed of analytical determination of water (moisture), crude fat (ether extract), crude protein and ash.
The seed samples were milled into flour using a pilot-plant hammer mill (Drotsky S1, Alberton, Gauteng, South Africa) with a 0.8 mm sieve. The flours were stored in airtight containers at 4°C until analysed. The moisture and fat content of the seed flours were measured by AOAC (Association of Official Analysis Chemists International) method No. 934.01 and AOAC method No. 920.39, respectively (AOAC, Citation2000). Crude ash content was determined by incinerating 2 g of the seed sample at 550°C in a muffle furnace, according to the AOAC method No. 942.05 (AOAC, Citation2010). Protein content (N X 6.25) was determined by the Dumas combustion analysis method, AACC (American Analytical Cereal Chemists International) method No. 46–30 (AACC, Citation2000) using a Dumatherm (DT, Gerhardt, Königswinter, Germany) instrument. All analyses were done in triplicate.
2.2.3. Mineral composition
The mineral content of the dried T. emetica, T. dregeana and soybean seeds were determined using the Agri-Laboratory Association of Southern Africa (AgriLASA) method (2007). The seeds were oven dried at 60°C for 24 h and milled using a Wiley mill to pass through a 1 mm sieve. The sample (0.5 g) was digested with 4 mL of 55% nitric Acid and 2 mL of 70% perchloric acid using an aluminium digestion block (Labcon D60 model) for 2 h at 100°C. The samples were further heated at 180°C for 6 h until decomposition of the organic substances. Deionized water was added to the cooled digested sample to make up to 25 cm3.
The micro-minerals [iron (Fe), zinc (Zn), copper (Cu), manganese (Mn) and boron (B)] and macro-minerals [calcium (Ca), magnesium (Mg), phosphorus (P), sulphur (S) and potassium (k)] were determined by Atomic Absorption Flame Spectroscopy and Flame Emission Spectroscopy (Varian AA200); with an Auto Analyser (Bran and Luebbe Auto Analyzer 3). The Ca, Cu, Fe, Mn, Mg and Zn were determined using AgriLASA method No. 6.5.1. The S, P, B and K were measured using AgriLASA methods No. 6.2.7.2; 6.3.1; 6.2.1.2 and 6.4.1, respectively. The analyses were carried out in triplicate. Mineral content was discussed as mg100−1g and content in 100 g of sample discussed as a percentage of the FAO/WHO recommended daily allowance (RDA) (FAO/WHO, 2004)
2.3. Light microscopic (LM) analysis
For LM, a single-edged razor blade was used to carefully remove the seed coat from each seed (n > 30 seeds). Each seed was separated into two cotyledons and the cotyledons were cut into 1 mm3 pieces. The cotyledons pieces were fixed using 0.15 M Sodium phosphate buffer, pH 7.2 containing 2.5% v/v glutaraldehyde/formaldehyde solution for 24 h. The samples were then washed three times with 0.15 M sodium phosphate buffer for 15 min each. Samples were dehydrated by using a graded series of ethanol (30%, 50%, 70%, 90% and 3x100%) for 15 min each. Resin embedding was done by using a graded series of LR White resin (SPI supplies) (20%, 40%, 60%, 80% and 100%) diluted in 100% ethanol, for 2 hours each, whilst samples were being rotated on an angled rotator. Resin embedding was done to preserve the tissue structure and the seed microstructure against mechanical and environmental damages. This was followed by four exchanges of fresh LR White resin, by leaving the samples at 4°C for 48 hours each. The resin was polymerized for 36 h at 60°C. The polymerized samples were sectioned (1 µm) using a Reichert Jung Ultracut E ultra-microtome. Samples were stained with toluidine blue and were observed under a Zeiss Axio Imager.M2 light microscope (Carl Zeiss, Germany) with the 20X, 0.45 NA and 40X, 0.75 NA objectives. The average size of the cells was measured from approximately 250 cells.
2.4. Confocal laser scanning microscopy (CLSM)
Freeze dried seeds were cut into sections using a single-edged razor blade and placed in an oven at 60°C for 36 h. Small tissue pieces (≤0.5 mm thick) were cut from the inner cotyledons by hand. The tissue pieces were stained with Nile Red (for lipid bodies) and Sulforhodamine 101 (for protein bodies) for 5 min. The stains were washed using 30% (v/v) glycerol solution. The stained sections were observed using a Zeiss LSM 880 confocal laser scanning microscope (Carl Zeiss, Germany), using a 10x, 0.3 NA objective. Excitation laser wavelength for both dyes was 561 nm, and the emission spectra in the range of 565 to 720 nm. A total of 20 lipid and protein bodies were captured.
2.5. Scanning electron microscope (SEM) analysis
The freeze-dried samples were dispersed in liquid nitrogen for 2 min and were sectioned longitudinally and in cross-sections. The samples were placed in a Petri dish and stored in an oven at 60°C for 36 h. Samples were coated with gold coating using EMITECH K550X (Quorum Technologies). The samples were observed under a Zeiss Ultra Plus Field Emission Scanning Electron Microscope (Carl Zeiss, Germany) at 500X, 1000X and 2000X magnification, with an accelerating voltage of 3kV and signal detected by a secondary electron detector. The average size of the cells was measured from at least 180 cells to provide statistical significance.
2.6. Image and statistical analysis
The ImageJ 1.43 u freeware software (National Institute of Health, Bethesda, Maryland, USA) was used to analyse all the images obtained from the microscopy techniques. Images were binarized (black and white) and three dimensions (length, width, and thickness) were measured.
Physical, proximate, and mineral analysis data were analysed by one-way analysis of variance (ANOVA) using Statistica® version 8 (Statsoft Inc, Tulsa). Size distribution data were tested for normality using the Kolmogorov-Smirnov and Shapiro-Wilk test. The mean values of physical, chemical, and structural analyses of T. emetica, T. dregeana and soybean seeds and flours were compared by the Fishers Least Significant Difference test (LSD) with a 95% confidence interval.
3. Results and discussion
3.1. The gross/external structure, physical and functional properties of T. emetica, T. dregeana and soybean seeds
The shape, appearance, and size of whole T. emetica and T. dregeana compared with soybean seeds observed under the stereo microscope are shown in . The T. emetica and T. dregeana seeds had a similar oblate shape and seed-coat colour (). Garnayak et al. (Citation2008), and Deshpande et al. (Citation1993) have observed that the spherical shaped seeds had a sphericity of above 0.8 and equivalent to sphere or oblate has a sphericity of <0.70. Both T. emetica and T. dregeana seeds were black and almost completely enveloped by a bright red aril while soybean seeds had a typical off-white to yellow seed-coat colour and were spherical in shape with a small hilum ().
Figure 1. Stereo microscopy images showing the external seed structure of T. dregeana (a), T. emetica (b) and Soybean (Glycine max (L.) Merrill) (c) seeds.
Figura 1. Imágenes de microscopía estereoscópica que muestran la estructura externa de las semillas de T. dregeana (a), T. emetica (b) y soya (Glycine max (L.) Merrill) (c)
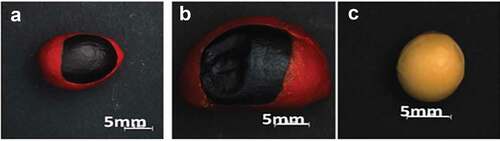
The T. emetica seeds which had an average size (Ferret’s diameter) of 39.38 ± 2.28 mm (aspect ratio: 1.72 ± 0.33, n = 30) whilst T. dregeana seeds had an average size of 24.95 ± 1.41 mm with an aspect ratio of 1.85 ± 0.00. The T. emetica and T. dregeana seeds were clearly larger than soybean seeds that had an average size of 13.94 ± 1.24 mm (aspect ratio of 1.17 ± 0.178) (). The aspect ratio (AR) of Trichilia spp indicated that the seeds were not a perfect round shape, while the soybean seeds had a more closely round shape.
The sphericity of T. emetica and T. dregeana seeds were not significantly different (p > .05). However, T. emetica and T. dregeana seeds had a significantly (p ≤ 0.05) lower sphericity than soybean (). The sphericity values for T. emetica and T. dregeana seeds are similar to the sphericity values for castor oilseeds (Pereas-Flores et al., Citation2011), but lower than the sphericity values for rapeseeds (0.91–0.93) (Sedat et al., Citation2005). In 2011, Pereas-Flores et al. reported that the castor oilseeds had a sphericity of 0.67 and stated that their results indicated that castor oilseed shape could cause difficulty to roll on surfaces and promotes sliding. The present results indicate that T. emetica and T. dregeana seeds, like the castor seeds, could be more cumbersome to handle compared with soybean, adjustment of soybean-based systems or design of appropriate systems may be required.
Table 1. Physical properties of Trichilia species and soybean.
Tabla 1. Propiedades físicas de las especies de Trichilia y de la soya
The geometric mean diameter for soybean were significantly (p ≤ 0.05) lower than T. emetica (). Rapeseeds were previously reported to have lower geometric average diameter (1.96–2.11 mm) (Unal et al., Citation2009) than soybean (12.6 mm), T. emetica (29.7 mm) and T. dregeana (16.9 mm). The geometric mean diameter is related to mass or energy transfer rates through the surface of the seeds (Niveditha et al., Citation2013). The GMD results of the current study suggest that T. emetica seeds have higher mass and heat transfer rates, which would more efficiently facilitate milling, cooling, drying, and heating operations compared with soybean.
The physical properties of T. emetica and T. dregeana seeds in comparison with the soybean are shown in . The true densities of soybean, T. emetica and T. dregeana seeds were not significantly different (p > .05). True density above 1000 kgm−3 suggests that the seeds are heavier than water and this can be used to design cleaning or separation equipment (Unal et al., Citation2009). The bulk density of T. emetica seeds was significantly (p ≤ 0.05) lower than that of T. dregeana (). Both T. dregeana and T. emetica seeds had significantly (p ≤ 0.05) lower bulk density compared with soybean (). Bulk density influences the storage of seeds (Unal et al., Citation2009) a higher bulk density is preferred in storage, packaging and transportation of food materials (Bhattachanya, Citation2011). Therefore, storage and packaging models used for soybeans seeds may not be appropriate for the Trichilia spp. However, the results suggest that the lower bulk density of T. emetica and T. dregeana seeds may be advantageous in drying, particularly in tunnel and fluidized bed drying. The air flow in the seeds could occur more rapidly than soybeans, which would imply shorter drying time and lower energy consumption relative to soybeans. Higher air flow rates and shorter drying times are important in minimising physiological damage to seeds (Maskan, Citation2000; Zhang et al., Citation2006). Hence, under the same drying conditions (temperature, relative humidity, and air speed), relatively more flavour compounds, colour and nutrients could be retained during drying of the Trichilia spp compared with soybeans seeds. This is further supported by the porosity results.
The T. dregeana had similar (p > .05) porosity, with a significantly (p ≤ 0.05) lower average unit volume compared with T. emetica. The porosity of the T. emetica and T. dregeana were however, significantly (p ≤ 0.05) higher than that of the soybean (). Porosity depends on geometry and surface properties of the material (Unal et al., Citation2009). The ratio of the empty seed space to its total volume is frequently needed in the airflow and heat flow studies (Mohsenin, Citation1986). This is used in the calculation of the rate of aeration and cooling, drying and heating, and the design of heat exchangers (Unal et al., Citation2009). The higher porosity values of these Trichilia spp seeds may be associated with irregular shape seeds and this suggest that the seeds have better aeration and water vapour diffusion during drying and storage when compared with soybeans. According to Adebowale et al. (Citation2011), based on studies involving moisture reconstituted seeds (10–30% moisture), higher moisture content was associated with higher porosity. On the other hand, the results of moisture content (<9.1%) in the present study () show that the Trichillia spp seeds had lower moisture content than that of soybeans which apparently contradicts the assertion that higher moisture content leads to higher porosity. The fact that the maximum moisture content in the present research (≤9.1%) was out of range of the values used by Adebowale et al. (Citation2011) (10–30%), probably explains the difference, given anticipated cohesion of seeds due to higher moisture content (>10%) would not occur in the present research. It may be noted that the equation for porosity (EquationEquation (2)(2)
(2) ), includes a ratio of bulk density to true density. Therefore, in the present study, the influence of the lower bulk density of the Trichilia spp seeds, due to their unique external dimensions compared with soybeans (), probably influenced the porosity more than the moisture content.
Table 2. Chemical composition of T. emetica, T. dregeana and Soybean flour.
Tabla 2. Composición química de T. emetica, T. dregeana y harina de soya
3.2. Nutritional composition of T. emetica and T. dregeana flour
The composition results of T. emetica, T. dregeana and soybean seeds are shown in . The crude protein content of T. emetica was significantly higher (p ≤ 0.05) than of T. dregeana but Trichilia spp seeds had significantly (p ≤ 0.05) lower protein content than soybean seeds (). The protein content for T. dregeana seeds obtained in the current study was lower than the protein content for canola seeds (23.6%) (Wanasundara, Citation2011). The protein content for T. emetica was within the range previously reported for sunflower seeds (20.4–40.0%), however at the lower range (Gonzalez-Peres & Vereijken, Citation2007) and canola seeds (23.6%) (Wanasundara, Citation2011). These results suggest that the two Trichilia spp are a comparable source of protein to common commercial oil seeds. However, further studies need to be conducted to identify the amino acid profile of the proteins and anti-nutrients in order ascertain their nutritional contribution and safety.
The crude fat content of T. emetica and T. dregeana were similar (p ≤ 0.05). The crude fat content of T. emetica and T. dregeana were in line with the crude fat content of other commercial sources of oil such as castor oilseed (33.8–56.2%) (Pereas-Flores et al., Citation2011; Vasco-Leal et al., Citation2018), and sunflower (30–36.8%) (Ingale & Shrivastava, Citation2011; Pereas-Flores et al., Citation2011). In the current study, the crude fat content for the soybean was significantly (p ≤ 0.05) lower than the crude fat content of T. emetica and T. dregeana (). The high crude fat content of the two seeds suggest that they can be a source of commercial oil. However, the fatty acid profile of the two seed oils needs to be assessed for further identification of potential uses. In addition, the effect of agronomic conditions, extraction conditions, and seed maturity on oil yield needs to be assessed. The ash content for the Trichilia spp and soybean seeds were not significantly different (p ≤ 0.05) (). Soybean seeds had significantly (p ≤ 0.05) higher moisture content compared with the Trichilia spp seeds (). Although the moisture contents were significantly different. They were all lower than 10%, which is the recommended level for long term storage of seed products (Wilson & Downs, Citation2012).
3.3. Mineral composition of T. emetica, and T. dregeana seeds
The mineral composition of T. emetica, T. dregeana and soybean is shown in . Potassium (K) was the most prominent macro-mineral in the two Trichilia spp seeds. The K content was decreased in Trichilia spp seeds in comparison with soybean. The higher K content of the T. dregeana suggests that it could be a better source of K for humans compared with T. emetica. Potassium helps in the maintenance of body fluids and electrolyte balance. It is involved in the proper function of the heart muscles and plays a role in carbohydrate metabolism and protein synthesis (Drewnowaki, Citation2010). The K content of T. dregeana and T. emetica seeds were higher than the previously reported for other oilseeds such as sunflower (0.067–0.075 mg.100 g−1) (Gonzalez-Peres & Vereijken, Citation2007) and peanut oilseeds (564–614 mg.100 g−1) (Jonnala et al., Citation2005). Cotton seeds and rapeseeds, however, have higher K content (1110 mg.100 g−1 and 1149–2232 mg.100 g−1; respectively) (Beyzi et al., Citation2019; He et al., Citation2013) than the values obtained in the current study for T. emetica. The T. dregeana seeds met 28.72% of K potassium RDA for adult men and 26.47% for adult women whilst T. emetica met lower values at 22.87% and 21.08% of the RDA for men and women, respectively. On the other hand, soybeans K RDA% coverage was higher than the Trichilia spp seeds at 36.06% and 33.24% for men and women, respectively.
Table 3. Mineral composition of T. emetica, T. dregeana and Soybean flour.
Tabla 3. Composición mineral de T. emetica, T. dregeana y harina de soya
Calcium was the second highest macro-mineral in both Trichilia spp. The Ca content was comparable in Trichilia spp but was significantly (p ≤ 0.05) higher than soybeans in the current study. The Trichilia spp seeds met higher percentages of the Ca RDA compared with soybeans. Trichilia emetica met 24.0–29.0% and T. dregeana met 25.0–30.0% of Ca RDA for both women and men, whilst soybean had covered 20.0–25.0%. Trichilia spp seeds were higher in Ca content than the previously reported oilseeds such as cottonseeds (145 mg.100 g−1) and soybean (74–83 mg.100 g−1) (Biel et al., Citation2018; He et al., Citation2013). Oil-seed derived non-dairy milk products such as soya milk, have to be supplemented with Ca due to their low Ca content (Chaiwanon et al., Citation2000). Non-dairy milk products from T. dregeana and T. emetica seeds could hence require less supplementation with calcium compared with soybean.
Iron and Zinc were significantly (p ≤ 0.05) lower in Trichilia spp seeds in comparison with soybeans (). The T. dregeana seeds met 79.1% and 23.4–35.2% of Fe RDA while T. emetica met about 85.4% and 25.3–37.9% of Fe RDA, for men and women, respectively. Both Trichilia spp had lower Fe RDA percentages than soybean which met 125.0% and 37.0–55.6% of Fe RDA for men and women, respectively. The T. dregeana had coverage of 20.9% RDA of Zn intake for men and 28.8% RDA of Zn for women compared with soybean with coverage of about 31.8% RDA and 43.8% RDA of Zn intake for men and women: respectively. Trichilia emetica had coverage of about 26.4% RDA and 36.3% RDA of Zn intake for both adult men and women. T. emetica seeds had significantly (p ≤ .05) higher Mg, Mn and Zn compared with T. dregeana. However, Trichilia spp seeds had significantly (p ≤ 0.05) lower Mg, Mn and Zn in comparison with soybean (See ). Magnesium plays an important role in the function of smooth muscle and cell production, while Mn and Zn are required by the immune system (Drewnowaki, Citation2010). Trichilia dregeana met 33.8–35.5% and 44.4–45.8% of Mg RDA for men and women respectively while T. emetica met a higher percentage of Mg RDA at 44.0–46.3% and 57.8–59.7% of Mg RDA adult men and women; respectively. Both Trichilia seeds met lower percentages of Mg RDA than soybeans which had 55.5–58.3% and 72.8–75.2% of Mg RDA for men and women, respectively. The T. dregeana seeds covered 100% RDA intake of Mn for women and 78.3% RDA intake of Mn for men. Soybean had Mn recommended daily intake coverage of about 165.5% for men and 212.8% for women. The T. emetica met about 108.7% and 138.9% of Mn RDA for adult men and women, respectively. These results imply that, in general, the T. emetica seed was a better source of these minerals (Mg, Mn and Zn) compared with T. dregeana seeds.
The T. emetica and T. dregeana seeds had significantly (p ≤ 0.05) lower phosphorus, sulphur and boron contents compared with soybean (See ). The T. emetica seeds met 47.09% of P RDA compared with 49.5% of P RDA met by T. dregeana for both adult men and women. Soybean met a significantly (p ≤ 0.05) higher percentage (84.2%) of P for both men and women. Trichilia spp had less coverage of recommended daily intake of P compared with cottonseeds and rapeseeds (Beyzi et al., Citation2019; He et al., Citation2013). The Trichilia spp seeds met >100% of the RDA of Cu and S. The T. emetica and seeds met 125.6% Cu RDA, whilst T. dregeana met 111.1% Cu RDA of Cu for both men and women and soybean met a similar (p ≥ 0.05) percentage of 118.9% of the Cu RDA. The sulphur RDA% met by T. emetica was 310.9% while that of T. dregeana 258.2% for both men and women while soybeans (667.3%) met a significantly (p ≤ 0.05) higher percentage of S RDA than both Trichilia spp seeds.
The lower K, Mg and P of the T. emetica and T. dregeana may be related to the lower crude protein content of the seeds. Protein body structures contain globoid inclusion bodies, which previously have been shown to contain high levels of P, K and Mg (Lott et al., Citation1982; Prego et al., Citation1998). The lower mineral composition of T. emetica and T. dregeana seeds compared with soybean is in accordance with previous reports on other oilseeds. The presence of higher or comparable levels of Fe, S, Mg, P, K and Zn relative to other oilseeds apart from soybean is an indication that the T. emetica and T. dregeana seeds may also serve as commercial oil seed food. The bioavailability of the minerals however needs to be assessed to ascertain their potential nutritional contribution.
3.4. Microstructure of Trichilia species
Seed micro-structure helps in understanding the spatial arrangement of identifiable elements such as proteins, starch, fibres, and lipids in food. Understanding the seed micro-structure can also improve the utilization of the seeds for different purposes (Aguilera, Citation2005; Prada & Aguilera, Citation2007).
Thin sections (<0.5 mm thick) of resin-embedded T. emetica, T. dregeana and soybean seeds stained with Toluidine Blue was used to observe the interior tissue cell structure, under light microscopy () In this study, T. emetica and T. dregeana seeds had ovoid-like parenchyma cells compared with soybean discoid cells (). Furthermore, Trichilia seed cell size and shape differed from those of soybean. The T. emetica had a slightly pinkish-purple staining colour compared with the T. dregeana and soybean seeds. This may suggest that the T. emetica seed has more carboxylated-polysaccharides compared with soybean seed (Mitra & Loque, Citation2014). However, further investigation for the presence or absence of carboxylated-polysaccharides has to be done. The T. emetica had significantly fewer large cells (48 cells per micrograph) compared with T. dregeana (100 cells per micrograph) with larger protein bodies (107.35 ± 93.56 µm) (Refer to the black lines on ). T. dregeana had smaller cells (48.20 ± 5.74 µm and AR of 1.54 ± 0.20) compared with soybean (85 cells, 63.27 ± 7.81 µm with aspect ratio of 1.84 ± 0.35) with smaller sized protein bodies (56.03 ± 16.90 µm) ().
Figure 2. Light microscopy showing the interior tissue cell structure of Trichilia emetica (a), Trichilia dregeana (b) and Soybean (c) seeds cells at 40X magnification. Protein bodies (PB) are stained blue with Toluidine blue. Scale Bar: 50 µm.
Figura 2. Microscopía de luz que muestra la estructura celular del tejido interior de las células de las semillas de Trichilia emetica (A), Trichilia dregeana (B) y soya (C) con un aumento de 40X. Los cuerpos proteicos (PB) se tiñen de azul con azul de toluidina. Barra de escala: 50 µm
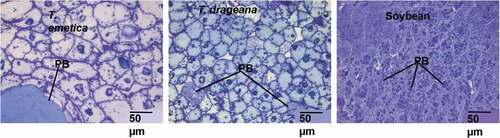
T. dregeana had more protein bodies per micrograph (6 per micrograph at 100 µm) compared with T. emetica (average 1 per micrograph) (). The protein body size for T. emetica and T. dregeana seeds were larger than the range previously reported for other oilseeds such as almond nuts with a protein body size of up to 12 µm and marama bean seeds of about 13 µm diameter (Mosele et al., Citation2011a; Young et al., Citation2004b). Trichilia emetica and T. dregeana had fewer and larger sized protein bodies compared with Soybean (100 ± 9 per micrograph; protein bodies: 13.08 ± 1.07 µm) (refer to the black lines on ). This could be due to the lower protein content for the Trichilia spp in comparison with the same component content in soybean seeds. The appearance of the purplish-blue-stained ovoid protein bodies was in agreement with other oilseed parenchyma cells such as castor seeds (Pereas-Flores et al., Citation2011) and almond nut (Ellis et al., Citation2004). Also, Ellis et al. (Citation2004) observed the presence of intracellular components (lipid and protein bodies) when almond nuts were stained with toluidine blue.
The microstructure of the seeds (Trichilia spp) examined under confocal laser scanning microscopy (CLSM) to further confirm the different components in them. The CSLM results for T. emetica and T. dregeana showed that the seeds had more oval shaped lipid bodies (size: 15.58 µm and 10.81 µm, respectively) compared with soybean discoid lipid bodies (10.41 µm) () as it was observed in BFLM and in proximate analysis section (fat content). The shape of the lipid bodies of seeds of Trichilia spp and soybean was similar to the shape of lipid bodies of castor oilseed (Pereas-Flores et al., Citation2011). However, the average sizes of peanut (1–3 µm) (Young et al., Citation2004a) and castor seed (12.63 ± 1.30 µm) (Pereas-Flores et al., Citation2011) were smaller than those of T. dregeana, T. emetica and soybean reported in the current study. These results implied that methods used for extraction of soybean oil, can be adapted for extraction of the Trichilia spp oil.
Figure 3. Confocal laser scanning microscopy showing the interior tissue cell structure of T. emetica (a,b) and T. drageana (c,d) compared with Soybean (e,f) cells. The protein bodies contain globoids inclusions (GI). The Nile red stained the lipid bodies (LB) and the sulfurhodamine 101 acid chloride stained the protein bodies (PB). G is the protein bodies with globoids inclusion at higher magnification.
Figura 3. Microscopía confocal de barrido láser que muestra la estructura celular del tejido interior de T. emetica (a,b) y T. drageana (c,d) en comparación con las células de soya (e,f). Los cuerpos proteicos contienen inclusiones globoides (GI). El rojo Nilo tiñó los cuerpos lipídicos (LB) y el cloruro ácido de sulfurhodamina 101 los cuerpos proteicos (PB). G representa los cuerpos proteicos con inclusión de globoides a mayor aumento
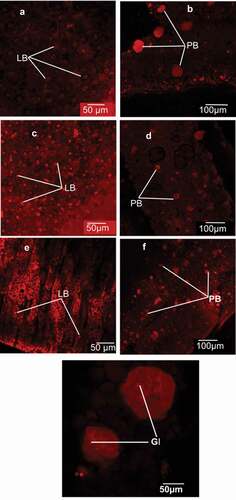
The protein (ovoid) body of T. emetica, T. dregeana and soybean differed in size (). This was similar to the protein bodies observed under bright field light microscope (). T. emetica had more, and larger, protein bodies (12 per micrograph; 144.3 µm) compared with T. dregeana (8 per micrograph; protein bodies 38.8 µm) (). Soybean had the smallest protein bodies (29.2 µm) when compared with T. emetica and T. dregeana (). These results implied that extraction of protein from the Trichilia spp, could be easier due to easier access to the larger protein bodies by the solvating agent. The variations in Trichilia spp and soybean protein body size and number may have contributed to the lower protein content of Trichilia spp and high protein content of soybean. At higher magnification, smaller spherical globoid inclusion bodies were observed within the larger protein bodies of Trichilia spp and Soybean seeds (). The spherical globoid inclusions within the protein bodies of the T. emetica and T. dregeana seeds were similar to oilseeds such as peanuts (Young et al., Citation2004a), marama bean (Mosele et al., Citation2011a) and castor seeds (Pereas-Flores et al., Citation2011). This implied that the similar methods for protein extraction for soybean seeds could be applied for Trichilia seed protein extraction.
The results obtained under BFLM and CLSM were as validated with SEM. The SEM showed that the parenchyma cells of Trichilia seeds contained protein bodies, which were embedded in a matrix of material that was reported for other oilseeds previously (). The presence of lipid and protein bodies in the parenchyma cells of seeds of both Trichilia spp (emetica and dregeana) agreed with previous observations made by Young et al. (Citation2004b) on almond nuts and Wroniak et al. (Citation2016) on rapeseeds. However, the SEM results for soybean showed that there were no protein and lipid bodies at low and high magnification (refer to ). This was probably due to the difference, in the response to the analysis methods for the soy sample whereby the light microscopy and CSLM staining facilitated observations, while SEM coating hindered the observation of soybean features.
Figure 4. Scanning electron microscope (SEM) showing the interior tissue cell structure of T. emetica (a), T. dregeana (b) and Soybean (c) seeds cells. LB: Lipid bodies and PB: Protein bodies. Scale Bar: 20 μm.
Figura 4. Microscopio electrónico de barrido (SEM) que muestra la estructura celular del tejido interior de las células de T. emetica (a), T. dregeana (b) y de las semillas de soya (c). LB: Cuerpos lipídicos y PB: Cuerpos proteicos. Barra de escala: 20 μm
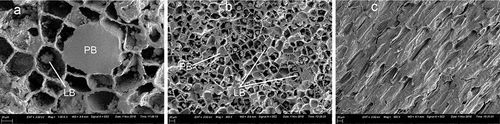
4. Conclusion
The present research successfully elucidated the gross structure, microstructure, physical characteristics, and nutritional composition of T. emetica and T. dregeana. The physical characteristics of the Trichilia spp indicated that modifications have to be made to existing soybean-based technologies for drying, packaging, and transportation in order to handle the Trichilia spp seeds. The protein, oil/fat, and mineral content results for Trichilia seeds suggested that the seeds could be a source of oil that could be viable for commercial uses, such as cooking oil, in cosmetics and fragrances, and for biodiesel production, but safety needs to be assessed first. The high calcium, protein, and oil contents of the T. emetica and T. dregeana could imply that they could be a source of non-dairy milk. The present research hence demonstrated the potential for commercial utilization of T. emetica and T. dregeana seeds; thereby leading to an increase in the number of available food sources for food insecurity alleviation, given they have comparable properties to existing commercial oil seeds. However, there is a need for further research to extensively assess the effect of agronomic conditions on their nutritional profile. These studies should include the bioavailability of minerals, fatty acid profiling, and the amino acid composition of the Trichilia spp proteins.
Author’s contributions
All authors contributed to the study as follows: Gugu Tsomele (experimental runs, data analysis, manuscript writing), Dr Obiro Cuthbert Wokadala (research conceptualization, experimental designs, student supervision, manuscript review), Dr. Elize Jooste (student supervision, manuscript review), Dr. Eudri Venter (microscopy analysis and manuscript review), and Dr Bhekisisa Dhlamini (proximate analysis and manuscript review), Dr. Nomali Ngobese (samples preparation, manuscript review), Dr. Mthulisi Siwela (student supervision, manuscript review). All authors read and approved on the manuscript for submission. The manuscript ID is TCYT-2020-0232
Geolocation information
Agricultural Research Council Tropical and Subtropical crops is situated at 25 27 04. 6S and 30 58 09. 1E co-ordinates in Mpumalanga, Mbombela.
Acknowledgments
The authors gratefully acknowledge funding from the National Research Foundation (NRF) (Grant Number 98687), Agricultural Research Council Tropical and Subtropical crops (ARC-TSC) and the University of KwaZulu-Natal for the support of this study. Mrs. Aylward Elsabe and Tshimangadzo Malindi are thanked for their technical support.
Disclosure statement
All the authors have no conflicts of interest to declare. The authors also have no relevant financial or non-financial interest to disclose.
Availability of data
All Authors can confirm that all relevant data are included in the article and/or its supplementary information files.
Additional information
Funding
References
- AACC. (2000). Official methods of analysis (10th ed.).American Analytical Cereal Chemists International.
- Adebowale, A. A., Sanni, L. O., Owo, H. O., & Karim, O. R. (2011). Effect of variety and moisture content on some engineering properties of paddy rice. Journal of Food Science and Technology, 48(5), 551–559. https://doi.org/https://doi.org/10.1007/513197-010-0173-3
- Aguilera, J. M. (2005). Why food microstructure. Journal of Food Engineering, 67(1–2), 3–11. https://doi.org/https://doi.org/10.1016/j.jfoodeng.2004.05.050
- AOAC. (2000). Official methods of analysis (7th ed.).Association of official analysis Chemists International.
- AOAC. (2010). Official methods of analysis (17th ed.).Association of official analysis Chemists International.
- Beyzi, E., Gunes, A., Beyzi, S. B., & Konca, Y. (2019). Changes in fatty acids and mineral composition of rapeseed (Brassica napus oleifea L) oil with seed sizes. Industrial Crops and Products Journal, 129, 10–14. https://doi.org/https://doi.org/10.1016/j.indcrop.2018.11.064
- Bhattachanya, K. R. (2011). Analysis of rice quality. Woodhead Publishing.
- Biel, W., Gaweda, D., Jaroszewska, A., & Hury, G. (2018). Content of minerals in soybean seeds as influenced by farming system, variety and row spacing. Journal of Elementology, 23(3), 1644–2296. https://doi.org/https://doi.org/10.5601/jelem.2017.22.3.1483
- Cafiero, C., Viviani, S., & Nord, M. (2018). Food Security measurement in a global context: The food insecurity experience scale. Measurement Journal, 116, 146–152. https://doi.org/https://doi.org/10.1016/j.measurement.2017.10.065
- Chaiwanon, P., Puwastein, P., Nitithamyong, A., & Sirichakwal, P. P. (2000). Calcium fortification in soybean milk and Invitro Bioavailability. Journal of Food Composition and Analysis, 13(4), 319–327. https://doi.org/https://doi.org/10.1006/jfca.1999.0854
- Deshpande, S. D., Bal, S., & Ojha, T. P. (1993). Physical Properties of Soybean. Journal of Agricultural Engineering Research, 56(2), 89–98. https://doi.org/https://doi.org/10.1006/jaer.1993.1063
- Drewnowaki, A. (2010). The nutrient rich food index helps to identify healthy affordable foods. The American Journal of Clinical Nutrition, 91(4), 1095S–1101S. https://doi.org/https://doi.org/10.3945/ajcn.2010.28450D
- Ellis, P. R., Kendall, C. W. C., Ren, Y., Parker, C., Pacy, J. F., Waldron, K. W., & Jenkins, D. J. A. (2004). Role of cell walls in the bio-accessibility of lipids in almond seeds. American Journal of Clinical Nutrition, 80(3), 604–613. https://doi.org/https://doi.org/10.1093/ajcn/80.3.604
- Garnayak, D. K., Prsdhan, R. C., Naik, S. N., & Bhatnagar, N. (2008). Moisture-dependent physical properties of Jatropha seeds (Jatropha Curcas L. Industrial Crops and Products Journal, 27(1), 123–129. https://doi.org/https://doi.org/10.1016/j.indcrop.2007.09.001
- Gonzalez-Peres, S., & Vereijken, J. M. (2007). Sunflower protein: Overview of their physicochemical, structural and functional properties. Journal Science of Food and Agriculture, 87(12), 2173–2191. https://doi.org/https://doi.org/10.1002/jsfa.2971
- He, Z., Shankle, M., Zhang, H., Way, T. R., Tewolde, H., & Uchimiya, M. (2013). Mineral Composition of Cottonseed is Affected by Fertilization Management Practices. Journal of Agronomy, 105(2), 341–350. https://doi.org/https://doi.org/10.2134/agronj2012.0351
- Huizar, M. I., Arena, R., & Laddu, D. R. (2020). The global food syndemic: The impact of food insecurity, Malnutrition and obesity on the healthspan and the COVID-19 pandemic. Progress in Cardiovascular Diseases Journal, 64, 105–107. https://doi.org/https://doi.org/10.1016/j.pcad.2020.07.002
- Ingale, S., & Shrivastava, S. K. (2011). Chemical studies of new varieties of sunflower (helianthus annuus) LSF-11 and LSF-8 seeds. Agriculture and Biology Journal of North America, 2(8), 1171–1181. https://doi.org/https://doi.org/10.5251/abjna.2011.2.8.1171.1181
- Jonnala, R., Dunford, N., & Chenault, K. (2005). Nutritional composition of genetically modified peanut varieties. Journal of Food Science, 70(4), S254–S256. https://doi.org/https://doi.org/10.1006/jfca.1999.0854
- Komane, B. M., Olivier, E. I. & Viljoen, A. (2011). Trichilia emetica (Meliaceae) – A review of traditional uses, biological activities and phytochemistry. Phytochemistry Letters Journal, 4(1), 1–9.
- Lott, V. N., Greenwood, J. S., & Vollmer, C. M. (1982). Mineral reserves in Castor beans: The dry seed. Plant Physiology Journal, 69(4), 829–833. https://doi.org/https://doi.org/10.1104/pp.69.4.829
- Maskan, M. (2000). Microwave/air and microwave finish drying of banana. Journal of Food Engineering, 44(2), 71–78. https://doi.org/https://doi.org/10.1016/S0260-8774(99)00167-3
- Mitra, P. P., & Loque, D. (2014). Histochemical staining of Arabidopsis thaliana Secondary cell wall elements. Journal of Visual Experiement, e51381(87), 1–11. https://doi.org/https://doi.org/10.3791/51381
- Mohsenin, N. N. 1970. Physical properties of plant and animal materials ISBN 9780677023007. Gordon and Breach Science Publishers.
- Mohsenin, N. N. (1986). Physical properties of plant and animal materials (2nd Edition Revised ed.). ISBN: 9781000122633. Gordon and Breach Science Publishers.
- Mosele, M. M., Hansen, Å. S., Hansen, M., Schulz, A., & Martens, H. J. (2011a). Proximate composition, histochemical analysis and microstructural localisation of nutrients in immature and mature seeds of marama bean (Tylosema esculentum) - an underutilised legume. Food Chemistry Journal, 127(4), 1555–1561. https://doi.org/https://doi.org/10.1016/j.foodchem.2011.02.017
- Niveditha, V. R., Sridhar, K. R., & Balasubramanian, D. (2013). Physical and mechanical properties of seeds and kernels of canavalia of coastal sand dunes. International Food Research Journal, 20(4), 1547–1554. http://www.ifrj.upm.edu.my/20%20(04)%202013/5%20IFRJ%2020%20(04)%202013%20Shridhar%20(086).pdf
- Pereas-Flores, M. J., Chanona-Perez, J. J., Garibay-Febles, V., Calderon-Dominguez, G., Terres-Rojas, E., Mendoza-Perez, J. A., & Herrera-Bucio, R. (2011). Microscopy techniques and image analysis for evaluation of some chemical and physical properties and morphological features for seeds of the castor oil plant (Ricinus communis). Industrial Crops and Products Journal, 34(1), 1057–1065. https://doi.org/https://doi.org/10.1016/j.indcrop.2011.03.015
- Prada, J., & Aguilera, J. M. (2007). Food microstructure affects the bioavailability of several nutrients. Journal of Food Science, 72(2), 21–32. https://doi.org/https://doi.org/10.1111/j.1750-3841.2007.00274.x
- Prego, I., Maldonado, M., & Otegui, S. (1998). Seed structure and localization of reserves in Chenopodium quinoa. Animal Botany Journal, 82(4), 481–488. https://doi.org/https://doi.org/10.1006/anbo.1998.0704
- Sadowska, J., Jelinski, T., Blaszczak, W., Konopka, S., Fornal, J., & Rybinski, W. (2013). The effect of seed size and microstructure on their mechanical properties and frictional behavior. International Journal of Food Properties, 16(4), 814–825. https://doi.org/https://doi.org/10.1080/10942912.2011.567430
- Sedat, C., Tamer, M., Huseyin, O., & Ozden, O. (2005). Physical properties of rapeseeds (Brassica napus oleifea L). Journal of Food Engineering, 69(1), 61–66. https://doi.org/https://doi.org/10.1016/j.jfoodeng.2004.07.010
- South African National Biodiversity Institute. (2004). Plantz Africa: Trichilia dregeana Sond. (Report Number: SA Tree Number 300). Kirstenbosch National Botanical Garden Publishers. http://pza.sanbi.org/trichilia-dregeana
- Unal, H., Sincik, M., & Izli, N. (2009). Comparison of some engineering properties of rapeseeds cultivars. Industrial Crops and Products Journal, 30(1), 131–136. https://doi.org/https://doi.org/10.1016/j.jfoodeng.2004.07.010
- Usman, A., Thoss, V., & Nur-e-alam, M. (2018). Isolation and identification of flavonoids components from Trichilia emetica whole seeds. Journal of Natural Products and Resources, 4(2), 179–181. https://doi.org/https://doi.org/10.30799/jnpr.062.18040201
- Van Wyk, B., Van Wyk, P., & Van Wyke, B. E. (2000). Photographic guide to trees of South Africa. Briza Publications. ISBN-13: 9781920217044. https://www.cabdirect.org/cabdirect/abstract/20023197171
- Vasco-Leal, J. F., Mosquera-Artamonov, J. D., Hernandez-Rios, I., Mendez-Gallegos, S. J., Perea-Flores, M. J., Pena-Aguilar, J. M., & Rodriguez-Garcia, M. E. (2018). Physicochemical characteristics of seeds from wild and cultivated Castor bean plants (Ricinus communis L). Ingenieria EInvestigacion, 38(1), 24–30. https://doi.org/https://doi.org/10.15446/ing.investig.v38n1.63453
- Vermaak, I., Katamou, G. P. P., Komane-Mofokeng, B., Viljoen, A. M., & Beckett, K. (2011). African seeds oils of commercial importance- cosmetic applications. South African Journal of Botany, 77, 920–933.
- Wanasundara, J. P. D. (2011). Protein of Brassicaceae oilseeds and their potential as a plant protein source. Critical Review in Food Science and Nutrition, 51(7), 635–677. https://doi.org/https://doi.org/10.1080/10408391003749942
- Wandkar, S. V., Ukey, P. D., & Pawar, D. A. (2012). Determination of physical properties of soybean at different moisture levels. International Agricultural Engineering Journal: CIGR J, 14(2), 138–142. https://cigrjournal.org/index.php/Ejounral/article/view/2081/1616
- Wilson, A. L., & Downs, C. T. (2012). Fruit nutritional composition and non-nutritive traits of indigenous South African tree species. South African Journal of Botany, 78(8), 30–36. https://doi.org/https://doi.org/10.1016/j.sajb.2011.04.008
- Wroniak, S. V., Rekas, A., Siger, A., & Janowicz, M. (2016). Microwave pretreatment effects on the changes in seeds microstructure, chemical composition and oxidative stability of rapeseed oil. Food Science and Technologies Journal, 68, 634–641. https://doi.org/https://doi.org/10.1016/j.lwt.2016.01.013
- Young, C. T., Pattee, H. E., Schadel, W. E., & Sanders, T. H. (2004b). The microstructure of almond (Prunus dulcis (Mill.) D.A Webb cv. ‘Nonpareil’) cotyledon. Leb-ensmittel-Wissenschaft und-Technologie, 37(3), 317–322. https://doi.org/https://doi.org/10.1016/j.lwt.2003.09.007
- Young, C. T., Pattee, H. E., Schandel, W. E., & Sanders, T. H. (2004a). Microstructure of peanut (Arachis hypogaea L. cv. NC 7) cotyledons during development. Leb-ensmittel-Wissenschaft und-Technologie, 37(4), 439–445. https://doi.org/https://doi.org/10.1016/j.lwt.2003.10.016
- Zhang, M., Tang, J., Mujumdar, A. S., & Wang, S. (2006). Trends in microwave-related drying of fruits and vegetables. Trends in Food Science and Technology Journal, 17(10), 524–534. https://doi.org/https://doi.org/10.1016/j.tifs.2006.04.011