ABSTRACT
The study evaluated the technical feasibility of directly using the mixture of the inedible fractions of vegetables collected in a wholesale market, for the recovery of a (poly)-phenolic extract with antioxidant capacity. First, the characterization of the leaves and stems of broccoli, celery, carrots, beetroots, cauliflower and cabbage was carried out. Subsequently, the technical feasibility of the recovery of antioxidant compounds from the mixture of these vegetables was analyzed, using a fractional factorial design tailored for screening of the process conditions. Finally, a freeze-dried powdered product was obtained from the extract previously concentrated by vacuum evaporation. The results showed that the product had a total phenolic content of 1752 ± 58 GAE/100 g and antioxidant capacity of 1020 ± 44 mg Fe2+/100 g of dry powder. These inedible vegetable wastes could be an attractive source of natural antioxidants to obtain high added-value products with diverse uses as food and feed techno-functional ingredients.
RESUMEN
El estudio evaluó la factibilidad técnica de utilizar de manera directa la mezcla de las fracciones no comestibles de hortalizas recolectadas en un mercado mayorista, para la recuperación de un extracto (poli)-fenólico con capacidad antioxidante. Primero, se realizó la caracterización de las hojas y tallos de brócoli, apio, zanahoria, betarraga, coliflor y repollo. Posteriormente, se analizó la factibilidad técnica de la recuperación de compuestos antioxidantes a partir de la mezcla de estos vegetales, mediante un diseño experimental fraccionado adaptado para la selección de las condiciones de proceso. Finalmente, se obtuvo un producto en polvo liofilizado a partir del extracto previamente concentrado por evaporación al vacío. Los resultados mostraron que el producto tuvo un contenido de polifenoles totales (TPC) de 1752 ± 58 GAE/100 g y una capacidad antioxidante (AOC) de 1020 ± 44 mg Fe2+/100 g de polvo seco. Estos residuos vegetales no comestibles podrían ser una fuente atractiva de antioxidantes naturales para obtener productos de alto valor agregado como alimento e ingredientes tecno-funcionales.
1. Introduction
The (poly)-phenolic compounds contribute to the antioxidant capacity of plant extracts, interrupting the generation cycle of new radicals by means of the donation of hydrogen atoms or electrons. The antioxidant property is also attributed to their ability to chelate metal ions which are involved in the production of free radicals (Fukumoto & Mazza, Citation2000).
Among the methods most used for the characterization of antioxidant properties of natural (poly)-phenol compounds there is one based on the 2,2-diphenyl-1-picrylhydrazyl radical (DPPH*) (Blois, Citation1958) and the so-called ferric-reducing antioxidant power (FRAP) (Benzie & Strain, Citation1996), which, respectively, quantify the free-radical accepting ability and the ferric-reducing ability of its extracts. Besides, it has been established experimentally the existence of a linear correlation between antioxidant capacity (AOC) obtained by various in vitro tests associated with electron or radical scavenging, and the total polyphenol content (TPC) (Huang & Lim, Citation2015).
A variety of foods of vegetable origin provide benefits beyond basic nutrition, due to the presence of phenolic compounds, which have properties such as long-term protection against cardiovascular diseases, chemoprotective activity, antioxidant, and anti-inflammatory power (Kooti & Daraei, Citation2017; Ninfali & Angelino, Citation2013). Currently, there is interest in making preparations that contain these (poly)-phenolic compounds for applications as techno-functional ingredients with natural antioxidant capacity. Additionally, as a consequence of the antioxidant, antimicrobial/antifungal, and techno-functional properties of the bioactive components in vegetable extracts, these have become promising natural ingredients for the manufacture of food packaging (Jafarzadeh et al., Citation2020); and the improvement of the oxidative stability and shelf life of edible oils and meat products (Alirezalu et al., Citation2020; Blasi & Cossignani, Citation2020). Moreover, there is background that shows that dietary supplementation of animal feed with (poly)-phenols has been a promising strategy to improve the meat quality of livestock, by preventing oxidative deterioration of lipids and protein. In the same way as these compounds positively affect human health, they also cause beneficial effects on animal health (Cimmino et al., Citation2018).
In recent years has been intensified the identification of vegetables, or parts of them, that contain (poly)-phenolic antioxidants, which promote health and prevent diseases (Saini et al., Citation2019). One of these is the Brassicaceae family, which includes a range of horticultural crops like cabbage, broccoli and cauliflower, among others, of which the sprouts, inflorescences, leaves, roots, seeds and stems are part of human diets (Cartea et al., Citation2011). Recently, the utilization of some inedible vegetable kitchen wastes, specifically broccoli leaves and stems to obtain alcoholic extracts that contain glycosylated flavonoids has been reported, with a predominance of the kaempferol, quercetin, and isorhamnetin flavonols (Pacifico et al., Citation2019). Domínguez-Perles et al. (Citation2010) studied the in vitro DPPH* scavenging capacity of extracts obtained from the residues of broccoli crops in greenhouses. The results indicate that the level of AOC in these residues is within the range of those obtained from the usually marketed edible parts (inflorescences). Other studies have established that the inedible parts of some vegetables like beetroot leaves (Biondo et al., Citation2014), carrot leaves (Leite et al., Citation2011), and chicory (Llorach et al., Citation2004); and outer stems and bracts of artichoke (Punzi et al., Citation2014) have a significant AOC to consider them interesting sources to obtain extracts.
During marketing in wholesale vegetable distribution markets, the non-edible parts are discarded and disposed off in sanitary landfills. In one of the largest Marketing Channels of the Metropolitan Region, Chile, ~100 tons of residues are sent to landfill every day, even though some of that amount could be recovered and/or valorized (Miranda, Citation2018). Those residues are partly composed of offcuts like leaves and stems, (Nasrin & Matin, Citation2018) which correspond to 25–30% of the vegetables (Ajila et al., Citation2010). The content of antioxidant compounds, phenylpropanoids, and flavonoids in these inedible fractions are similar or even greater than the parts marketed for consumption (Feduraev et al., Citation2019; Huang & Lim, Citation2015). The exploitation of these kind of residues for the recovery of (poly)-phenols is an approach that leads to a greater added-value product with respect to other alternatives such as animal feed or composting (Angulo et al., Citation2012; Devkota et al., Citation2014).
Due to their high moisture content, vegetable wastes are prone to microbial spoilage which could cause some difficulties during storage. Consequently, it is necessary to include a pretreatment, thermal or non-thermal, to reduce their moisture and so, to preserve the phytochemicals they contain (Majerska et al., Citation2019; Sagar et al., Citation2018).
According to this point of view, it is necessary to implement an efficient technology for the recovery of natural antioxidants from wastes of fresh vegetables that are generated during their commercialization in a wholesale market. The present paper is focused on the direct utilization of the mixture of the inedible fractions of vegetables. The use of these heterogeneous mixtures of stems and leaves of various vegetables is scarcely reported in literature, so the present study was carried out at laboratory scale.
Solid-liquid extraction is still used in the recovery of natural antioxidants, although it is not the most efficient method available today. Other technologies with greater technical-economic feasibility, such as those called advanced, to date have not reached a significant development at an industrial level (Santos et al., Citation2020). The type of solvent and its polarity have an important role in the yield of (poly)-phenol extraction depending on the particularities of its structure (Pang et al., Citation2014). Therefore, setting the proper operational conditions such as particle size, temperature, solid–liquid ratio, solvent kind, and concentration would lead to improved efficiency of polyphenol extraction. Likewise, to get the best recovery yields of antioxidant compounds, including (poly)-phenols, during vegetable waste processing, an appropriate selection of methods and operational parameters was required (Fierascu et al., Citation2019).
In this framework, the objective of this work was to evaluate the technical feasibility of directly using the mixture of the inedible fractions of vegetables, as raw material to recover a (poly)-phenolic extract with antioxidant capacity.
2. Materials and methods
2.1. Inedible fractions of vegetables (IFV)
The inedible fractions of vegetables (IFV) were collected in the wholesale market (Santiago, Chile). To avoid possible analytical interferences mainly by salts such as carbonates, the IFV were washed with distilled water. Then, the IFV were spread out over grates, frequently turning them around to drain residual wash water by contact with air in ambient conditions (24 ± 4°C and 40 ± 10% humidity) during 4 h. Finally, the IFV were stored in vacuum sealed polypropylene bags at −20°C. shows images of the collected residues, leaves and stems of vegetables, specifically of celery (Apium graveolens), broccoli (Brassica oleracea var. italica), carrot (Daucus carota L.), beetroot (Beta vulgaris var. crassa), cauliflower (Brassica oleracea var. botrytis) and cabbage (Brassica oleracea var. capitata).
2.2. Chemicals
Gallic acid (≥99.5) and TPTZ (2,4,6-tri(2-pyridyl) S-triazine) were purchased from Sigma-Aldrich. Ethanol solvent (≥99.5) and Folin-Ciocalteu phenol reagent (FCR) were purchased from Merck. Sodium carbonate, sodium acetate trihydrate, potassium persulfate, glacial acetic acid, hydrochloric acid, ferric chloride hexahydrate, and ferrous sulfate heptahydrate were supplied by Soviquim Ltda with analytical grade.
2.3. Characterization of the inedible fractions of vegetables (IFV)
The IFV collected in the wholesale market were characterized by means of moisture content, ash content, total phenolic content (TPC) and antioxidant capacity (AOC).
The analyses were realized in triplicates, and results were expressed as mean ± standard deviation. An analysis of variance (ANOVA) was applied to determine the significant differences between different vegetables for each parameter (p < 0.05).
2.3.1. Determination of moisture and ash contents
The moisture content of the fresh samples was determined gravimetrically at 105°C in an oven (mod. U75, Memmert) according to the standard method (AOAC 925.09). The ash content of the samples was determined by calcination in a muffle furnace (mod. JMS-30 H, JRS) according to the standard method (AOAC 942.05). These were expressed as % wet base (g water/100 g) and (g ash/100 g), respectively (AOAC, Citation2006).
2.3.2. Extraction procedure from IFV to determine TPC and AOC
The extraction process was carried out according to the method used by Gonzales et al. (Citation2014). The frozen samples of IFV (3.5 g) were manually cut into 50 mm chunks and then were triturated with an electric chopper during a standardized time of 1 minute (Chopper, Moulinex). The chopped samples were added to flasks containing 35 mL of ethanol/water 65/35 solvent and then were placed in an orbital shaker with temperature control (Lab Tech, LSI-3 016 R). The residue-extractant contact was kept at 150 rpm and 30°C for 2 h. The extract was recovered by centrifugation at 2400 g for 10 min (IEC, Spinette). The supernatant liquid was collected in 15 mL centrifuge tubes covered with aluminum foil which were stored at −20°C, with the exception of those samples used immediately in their characterization that were kept at 4°C.
2.3.3. Determination of total (poly)-phenolic content
The total (poly)-phenolic content was quantified following the Folin-Ciocalteu method at a wavelength of 765 nm, using a UV-spectrophotometer (Rayleigh-UV-160) (Singleton et al., Citation1999). The amount of total phenol content was expressed as mg gallic acid equivalent per 100 g of fresh weight (mg GAE/100 g FW).
2.3.4. Antioxidant capacity assay
The AOC was quantified following the FRAP method at a wavelength of 765 nm (Benzie & Strain, Citation1996), using UV-spectrophotometry (Rayleigh-UV-160). The AOC was expressed in mg Fe2+ reduced per 100 g of fresh weight (mg Fe2+/100 g FW).
2.4. Determination of operational conditions to obtain antioxidant (poly)-phenolic extracts from IFV mixture
The determination of the operational condition to obtain antioxidant (poly)-phenolic extracts from IFV mixture was carried out using screening experimental design, which is detailed below.
2.4.1. Preparation of the mixture IFV samples
The IFV samples used to carry out the runs of the experimental design, consisted of a mixture of stems and leaves of the different vegetables in the following proportions: celery 25%, broccoli 25%, carrots 5%, beetroot 5%, cauliflower 25%, and cabbage 15%. The stem-to-leaf ratio was 2/3, 3/2, 2/3, 1/1, 1/0, 1/0 for celery, broccoli, carrots, beetroot, cauliflower and cabbage, respectively; these proportions are representative of the vegetable wastes generated in the wholesale market. The IFV mixtures were stored in vacuum sealed polypropylene bags and kept at −20°C.
For experimental tests, 30 g of these IFV mixtures was manually cut into 50 mm chunks and then triturated with an electric chopper during a standardized time of 1 minute (Chopper, Moulinex brand). Then, 3.0 g of FIV mixture and solvent was added to an Erlenmeyer flask (100 mL) at the conditions described in the experimental design ().
Table 1. Factors and levels used in 26−2 fractional factorial design.
Tabla 1. Factores y niveles utilizados en el diseño factorial fraccionado 26−2
2.4.2. Experimental design
The fractional factorial design (FFD) with resolution IV, 26−2, was used to quantify and rank the effect of the selected factors and their interactions in the (poly)-phenolic compounds extraction process from the IFV mixture, using the Statgraphics Centurion XVI software (Manugistics Inc., Rockville, MD). The use of FFD allows to reduce the number of experiments under the assumption that the interactions between three or more factors are of low significance compared to the effect of the main factors and of the interactions between two of them.
Six factors and two responses were selected, a total of 16 experiments were performed in duplicate, also including one central point in quadruplicate. The selected factors were temperature (X1), solvent composition (ethanol/water) (X2), solid/liquid ratio (X3), pH (X4), stirring speed (X5), and extraction time (X6), with two levels, namely, low (-) and high (+). The responses studied were TPC (Y1) and AOC (Y2). The details of experimental design are summarized in .
The main factors and their interactions were ranked according to the level of significance expressed as p value, accepting those that satisfy the condition p ≤ 0.05. A multiple regression analysis was performed obtaining a reduced regression model which corresponds to the first-order response function, which involved only the significant factors. The reduced regression model was formulated after examining the statistical significance of each regression coefficient, which was performed using the Student’s t-test at 5% significance level. The estimation of these regression coefficients corresponding to the best fit of the experimental data was carried out by the least squares method using statistical indicators. The coefficient of determination (R2) and the adjusted coefficient of determination (Adj. R2) were calculated to check the model adequacy. Once the extraction feasible conditions were selected, the reproducibility of the process was studied by triplicate.
2.5. Obtaining concentrated (poly)-phenolic extracts and powdered product from IFV mixture
The crude extract of the (poly)-phenolic compounds was obtained from 84 g of IFV mixture. This sample was contacted with 420 mL of solvent at the extraction conditions determined in the previous point. The crude extract was recovered by vacuum filtration through Whatman N° 1 filter paper, and concentrated by evaporation under vacuum conditions (Rotary evaporator LabTech, Model IV400) operated at 55°C, 70 kPa during 30 min. Finally, the concentrated extract was frozen at −20°C for 12 h and then freeze-dried (Labconco FreeZone 4.5 L lyophilizer, USA), at 0.28 mbar and −50°C during 24–36 h. The dry powder was stored at −20°C for further characterization.
3. Results and discussion
3.1. Characterization of the individual components of IFV
The moisture and ash content, together with the TPC and AOC values, are shown in . It is observed that the IFV have a moisture content that varies between 77.4% and 93.1% (w.b.). According to the bibliographic backgrounds, these high values of water content is one of the factors responsible for the turgor pressure inside the cell of vegetables and therefore, of its appearance and texture (Barrett et al., Citation2010). As to the ash content, it varies between 1.36% and 3.67%, a range expected for foods of plant origin (Khedkar et al., Citation2017).
Table 2. Characterization of leaves and stems of the inedible fractions of vegetables.
Tabla 2. Caracterización de las hojas y tallos de las fracciones no comestibles de los vegetales
In relation to the TPC, it is established that broccoli and carrot leaves have an average TPC 1.7 times greater than celery leaves, cauliflower, and cabbage; additionally, four times greater than beetroot leaves. Similarly, broccoli stems have a TPC that is 1.3–2.0 times greater than celery, carrot, and beetroot stems. Furthermore, the leaves have a TPC up to five times greater than the stems.
The selection of a suitable in vitro assay to evaluate the IFV mixture as a source on natural antioxidants, and the effect of the processing conditions of these sources on the antioxidant compounds recovery, was based on a previous research (Apak et al., Citation2016). This way, it was taken into consideration that to analyze samples with background color, like the case of IFV extracts, those methods that use colorimetric reagents whose chromophores absorb in the visible range of the spectrum, such as FRAP and DPPH, are recommended. Nevertheless, the content of any pigment in the samples causes greater adverse effects on the precision of methods based on color decomposition reactions, such as DPPH, than of those based on color formation reactions such as FRAP. Consequently, it was established that an assay using out sphere electron transfer agents such as FRAP may be the most suitable.
The AOC values of the different vegetables vary between 13.7 and 176.2 mg Fe2+/100 g FW, with a much greater presence in leaves than in stems. Likewise, the highest TPC together with AOC were obtained for broccoli leaves, followed by carrot leaves; and the lowest values were those of the outer leaves of cabbage and cauliflower. The content of polyphenols in the different parts and organs of each plant vary according to the function that these compounds fulfill in their life cycle, which has been reported for broccoli and plants of the Rumex L genus (Feduraev et al., Citation2019; Huang & Lim, Citation2015). In particular, for Rumex crispus and Rumex obtusifolius, it was reported that the TPC in decreasing order is seeds>flowers>leaves>roots>stems, because the leaves and flowers are subjected to greater exposure to solar radiation and air temperature, together with the probability of pathogen attacks, stimulating the synthesis of secondary metabolites as a response to environmental stress.
It has been reported a high positive correlation between the values of the TPC and AOC contents of leaves and stems from different varieties of celery and broccoli (Domínguez-Perles et al., Citation2010; Huang & Lim, Citation2015; Yao & Ren, Citation2011). In our study, it has been established that the values of TPC and AOC obtained from the individual components of IFV showed very high positive linear correlation which Pearson’s coefficients correspond to 0.95 (data not shown), indicating that the recovered (poly)-phenolic compounds are the main contributors to their antioxidant capacity.
The results obtained for the different vegetables are consistent with some publications, where the major (poly)-phenolic compounds that contribute to their antioxidant potential were besides identified. Summarizing, it was established that in carrot leaves the TPC values fluctuate between 170 and 404 mg GAE/100 g FW, detecting the presence of chlorogenic, neochlorogenic, quinic, sinapic, o-coumaric, and rosmarinic acid, in addition to flavonoids like quercetin and kaempferol (Aires et al., Citation2017; Alves et al., Citation2017; Burri et al., Citation2017). The leaves of beetroots were also reported as important sources of polyphenols and flavonoids, the gallic and rosmarinic acids, and quercetin are the major (poly)-phenolic compounds (Alves et al., Citation2017; Goyeneche et al., Citation2020; Ninfali & Angelino, Citation2013).
Celery is a vegetable whose stems and leaves are consumed with medical purposes; its effects over cardiovascular diseases, diabetes, and cancer have been studied (Kooti & Daraei, Citation2017). TPC values have been reported mostly in their stems, between 34.6 and 26.0 mg GAE/100 g FW, associated with p-coumaric, ferulic, and caffeic acids, together with flavonoids like apigenin, luteolin, and kaempferol (Yao & Ren, Citation2011).
For different varieties of broccoli, cabbage, and cauliflower, high values of TPC and AOC have been reported from their inedible parts as well as from their outer leaves. Huang and Lim (Citation2015) reported in water-methanol extracts (80%) of broccoli leaves and stems, TPC values between 196.5 and 80.5, and 62.8 and 411 mg of GAE/100 g FW, respectively. Likewise, for different cabbage varieties, high values between 153.9 and 86.6 mg GAE/100 g FW (Liang et al., Citation2019) were obtained, while a value of 167.7 mg GAE/100 g FW was obtained for cauliflower leaves (Babbar et al., Citation2014). Furthermore, for this type of vegetables the most abundant phenolic compounds that have been reported are sinaptic and ferulic acids. Additionally, the flavonoids that provide them their high AOC are kaempferol and quercetin (Gonzales et al., Citation2014).
All of the above means that the (poly)-phenolic compounds (phenolic acids and flavonoids) present in the leaves and stems of the vegetables have the ability to donate hydrogen atoms or electrons and eliminate free radicals, so they can therefore represent an important source of natural antioxidants and an attractive alternative of valorization for these types of residues. This result would allow the formulation of concentrated extracts that could have very beneficial effects on people’s health. It has been widely reported that vegetables have a complex polyphenols mixture characteristic of each species, giving it certain antioxidant capacity. The simultaneous consumption of various vegetables causes an additive AOC effect, which could be modified by means of synergistic or antagonistic interactions between the (poly)-phenolic compounds present in the mixture (Wang et al., Citation2011). It is therefore relevant to evaluate the TPC and AOC of the extracts obtained from the IFV mixtures. The total antioxidant capacity, measured as the thermodynamic conversion efficiency of reactive species by antioxidants, is indicative of the collaborative, summative action and of possible synergy/antagonism, of all the compounds of this type that are present in a complex sample (Apak et al., Citation2016). Hence, this type of measurement is considered a more useful parameter to evaluate the antioxidant properties of vegetables or food in general than the separate determination of the concentrations of the individual antioxidant components.
Exploring this IFV valorization alternative requires the determination of the feasible conditions of extraction that would allow to recover and concentrate the (poly)-phenolic compounds and that in addition, the loss of the antioxidant properties is as low as possible.
3.2. Fractional factorial design and determination of extraction conditions
3.2.1. Effects of the operational conditions on the extraction process
presents the design matrix for FFD and the responses together with the values of each of the levels. The FFD considered the value “without agitation” as the lowest level for the stirring parameter because within conventional extraction methods frequently the raw material is in contact with a specific solvent for a period of time, under this condition. The extraction of the (poly)-phenolic compounds was made from the IFV mixture consisting of stems and leaves. The extracts with the largest TPC and AOC correspond to experimental rows 1, 5 and 10, whose values vary between 126 and 131 mg GAE/100 g FW and between 61 and 77 mg Fe2+/100 g FW, respectively. These values are lower than those obtained from the discarded leaves of vegetables () which may be attributed to the stem content present in the mixture (an average of 50%). Furthermore, the AOC value estimated from the values of stems and leaves weighted according to the composition of the IFV mixture is 63 mg Fe2+/100 g FW, a comparable value to those obtained under some experimental conditions, showing a probable inexistence of interaction like synergy or antagonism between the (poly)-phenolic compounds present in the extract (Wang et al., Citation2011).
Table 3. Design matrix for 26−2 fractional factorial design with the observed responses for Y1-TPC and Y2-AOC.
Tabla 3. Matriz de diseño para diseño factorial fraccionado 26−2 con las respuestas observadas para Y1-TPC e Y2-AOC
The IFV mix extracts also exhibited a high positive correlation between the TPC and AOC values (Pearson’s coefficient of 0.94, showing once again that the main contributors to the antioxidant capacity of the extracts are phenolic compounds) (data not shown).
) show the standardized Pareto diagrams, where it is seen that the stirring speed, temperature, and the extraction time are the main factors that most significantly affect both responses (Y1-TPC and Y2-AOC). Effect of lower magnitude is produced by the solid/liquid ratio and pH, which are statistically significant only for Y1. Finally, in the working range, the solvent concentration does not produce effect with statistical significance on the Y1 and Y2 responses. It is also seen that the stirring speed, temperature, extraction time, solid/liquid ratio cause a positive effect on the extraction of (poly)-phenolic compounds with antioxidant capacity, while the solvent concentration and pH produce a negative effect.
Figure 2. Pareto chart (p < 0.05) of the significance categorized of standardized effects of extraction process main factors and its interactions on the responses (a) Y1-TPC: Total (poly)-phenolic content. (b) Y2-AOC: Antioxidant capacity.
Figura 2. Diagrama de Pareto (p < 0.05) de la categorización de significancia de los efectos estandarizados de los factores principales del proceso de extracción y sus interacciones sobre las respuestas. (a) Y1-TPC: Contenido (poli) fenólico total. (b) Y2-AOC: Capacidad antioxidante (a) Y1-TPC: Contenido de polifenoles totales. (b) Y2-AOC: Capacidad antioxidante
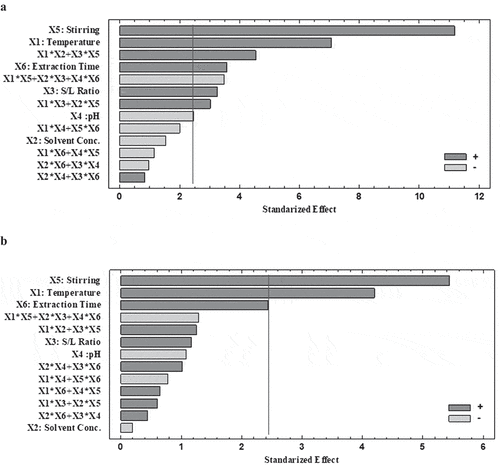
The (poly)-phenolic compounds are secondary metabolites that are stored in the vacuoles located inside of the plant cells. These vacuoles occupy 80–90% of the adult cells of leaves, stems, and roots (Roytrakul & Verpoorte, Citation2007). Therefore, to promote the transport of the (poly)-phenolic compounds from their storage points, it is required to break the walls to accelerate diffusion across them. A fast suspension stirring allows to speed up the emptying of the vacuoles by the transport through the membrane to the liquid medium that explains the significance of this variable in establishing the hierarchy of the effects. In the conventional batch extraction, this phenomenon of mass transfer occurs due to the concentration gradient of the antioxidant compounds from inside the solid to the liquid phase bulk. This operation takes place in unsteady-state since both antioxidant concentrations vary continuously. The mass transfer rate decreases as the concentration of the active compounds in the solvent increases, until equilibrium is reached. Consequently, the operation consists of two stages, a rapid extraction, so-called the washing stage, and a slow extraction known as the diffusion stage (Bengardino et al., Citation2019). Consequently, the process kinetics is affected by two resistances that act in series: the diffusion at the inside of particles, and the convective transport from the interface on the particles surface to the liquid phase bulk. As the process progresses, the concentration of the active compounds increases at the interface, while simultaneously decreases inside the solid, being the diffusion inside the particle the controlling stage. Thus, a high speed of agitation of the suspension favors the transport process, preventing the active compounds concentration from increasing steadily in the interface.
It has been reported that temperature has an influence on the extraction process of polyphenols from different plant matrices, because it has a favorable effect on the solubility and diffusivity of the polyphenol molecules, improving mass transfer from the inside of the plant matrix toward the bulk of the extractant liquid (Cuevas-Valenzuela et al., Citation2014; D’Alessandro et al., Citation2012; Pradal et al., Citation2016). Furthermore, it has been established that at higher temperatures the bonds of the phenolic compounds with the plant matrix are weakened, affecting the structure of the cell membrane and facilitating the release of the insoluble molecules (D’Alessandro et al., Citation2012). However, it must be kept in mind that these molecules are thermo-labile, so their stability decreases as the extraction temperature increases.
The significant effect of extraction time is probably due to the wide range chosen for the variable, 30–210 minutes, which includes the fast and slow stages of the extraction process (Bengardino et al., Citation2019). It should be pointed out that the amount of extracted polyphenols increases continuously over time, initially at a high extraction rate which decreases as the concentration approaches asymptotically the equilibrium value. It is for the same reason that some studies have reported that the extraction time turns out to be a not significant variable for high time ranges (Cujic et al., Citation2016). There are data that show that the highest concentration gradients, and therefore the highest mass transfer rates occur within the first 40 minutes of contact (Bucic-Kojic et al., Citation2007). It has also been reported that longer extraction times can cause a performance decrease because oxidation of the (poly)-phenolic compounds takes place, reducing the TPC and AOC of (poly)-phenolic compounds (Shi et al., Citation2003).
The solid/extractant solvent ratio is a variable that affects the efficiency of the process, which depends on the extractive ability of the solvent associated with the saturation and humectation of the solid. Large volumes of solvent increase the extracting capacity, but the concentration of the polyphenols contained in the liquid phase is reduced, with the consequent increase in energy cost in the concentration process. Some authors have reported results using large solvent volumes (1/10-1/50 g/mL) (D’Alessandro et al., Citation2012; Pradal et al., Citation2016) which agree with those obtained in the present work.
The pH is a factor that, although it can affect the polyphenolic compounds extraction yield and its properties, it has not been sufficiently reported. Ahmed et al. (Citation2020) reported that as the solvent acidity increases, gradual improvements in total phenolic content and antioxidant capacity are obtained. When the extraction is carried out at values lower than physiological pH (pH = 7) the solubilization of phenolic compounds and their diffusion from vegetable cells can increase, which is associated with higher extraction yields. Likewise, there is evidence that natural phenolic compounds display better stability under acidic conditions (<pH 6).
The chosen solvent was an ethanol/water mixture used for the extraction of polyphenols, due to certain advantageous properties of this solvent such as low toxicity for higher life forms and significant evaporation rate at low temperature, therefore low energy consumption. According to literature data, the variation of the ethanol/water composition of the mixture affects the dielectric constant (polarity) of the solvent and the solubility of the (poly)-phenolic compounds. This has been reported by Cuevas-Valenzuela et al. (Citation2014), who determined that an increase in the proportion of ethanol from 0% to 13% increases the solubility of the catechin molecules more than five times. In contrast, D’Alessandro et al. (Citation2012) and Pradal et al. (Citation2016) reported that an increase of ethanol in the solvent’s composition from 20% to 50% slightly improved the extraction of (poly)-phenolic compounds from berries (Aronia melanocarpa) and from byproducts of chicory. This agrees with the results obtained in the present work, where the effect of solvent composition was not significant. Furthermore, it has been reported that the solvent which composition is greater than 50% ethanol has lower capacity for extracting (poly)-phenolic compounds from the plant matrices (Pradal et al., Citation2016).
3.2.2. Determination of technically feasible extraction conditions
shows the statistical significance p-values, for the main effects and second-order interaction terms. In addition, the coefficients of the models are presented. In the case of the Y1-TPC response, the main factors temperature (X1), solid/liquid ratio (X3), pH (X4), stirring speed (X4) and stirring time were considered for the model. In addition to the X1 * X3, X1 * X5 and X3 * X5 interactions. On the other hand, the coefficients of the regression model of the Y2-AOC response were temperature (X1), stirring speed (X5) and extraction time (X6). The R2 correlation coefficient values were 98.8% and 95.4%, for the models adjusted to the Y1-TPC and Y2-AOC responses, respectively. In addition, the differences between R2 and (adjusted) R2 of 3.7% and 14.0%, for both responses, imply that the factors considered for the model can represent the response satisfactorily.
Table 4. Coefficients of the regression model for the Y1-TPC and Y2-AOC.
Tabla 4. Coeficientes de regresión del modelo para Y1-TPC y Y2-AOC
By means of the proposed model, it was determined that the conditions for the highest extraction of polyphenols are a temperature of 55°C, 40% ethanol solvent concentration, 1/5 (g/mL) solid/liquid ratio, pH 3, 200 rpm stirring speed, and 210 min of stirring time, predicting a TPC value of 144 mg GAE/100 g FW. On the other hand, the operating conditions that allow to obtain extracts with the largest AOC are the following: temperature 55°C, 20% ethanol solvent concentration, 1/5 (g/mL) solid/liquid ratio, pH 7, 200 rpm of stirring speed, and 210 min of extraction time; the extract presented an AOC of 71.8 mg Fe2+/100 g FW.
Finally, according to the cost-benefit criteria the technically feasible extraction conditions selected for the recovery polyphenols with an antioxidant ability from IFV mixtures were: a stirring speed of 100 rpm, because the mean agitation level instead of the predicted does not reduce significantly the recovery of polyphenols, a temperature of 55°C, a 60 min extraction time had allows recovering 80–90% of the soluble compounds, solid/liquid ratio of 1/5 g FW/mL that allows to obtain more concentrated extracts, a pH of 3, and a 20% ethanol solvent concentration, which turned out to be a factor of little significance and economically more favorable.
In the confirming tests for the validation of the model, the extracts presented a TPC of 98.3 ± 1.8 mg GAE/100 g FW, whose concentration in the extract was 196.7 ± 3.7 mg GAE/L. In turn, the AOC of the polyphenols present in the extract was 56.0 ± 1.6 mg Fe2+/100 g FW. The values were within the 95% confidence of the models’ prediction (90–107 mg GAE/100 g FW and 49–60 mg Fe2+/100 g FW).
3.3. Production of concentrated extract and freeze-drying powder
The results of the inventory of the IFV valorization process are shown in . It is observed that the crude extract volume (CRE) is equivalent to 90.5% of the fresh solvent. In the next stage of concentration by vacuum evaporation, the solvent extractant was reduced in 40.8%, obtaining an intermediate product which was called concentrated extract (CE). Also, in this concentration stage both (poly)-phenolic content and antioxidant capacity were increased by 50%. Finally, 3.23 g of the dehydrated extract that was obtained from the freeze-drying stage represents 27.9% of the dry weight of IFV. Images of the crude and concentrated extract, as well as powdered product, are shown in . It can be seen that a pinkish-red coloration is exhibited due to the betalains content extracted from beetroot leaves. The concentration factors, determined by the ratio of the content of polyphenols and antioxidant capacity between the powdered product and the mixture of inedible fractions of vegetables (IFV), were 18.7 and 20.8, respectively.
Table 5. Characterization of intermediate products and final product (powder) of the phenolic antioxidant recovery process from IFV.
Tabla 5. Caracterización de productos intermedios y producto final (polvo) del proceso de recuperación de antioxidantes fenólicos a partir fracciones no comestibles de vegetales
Figure 3. Extracts obtained from the mixture of inedible fractions of vegetables. (a) Crude extract (b) Concentrated extract (c) Dehydrated extract (powder).
Figura 3. Extractos obtenidos de la mezcla de las fracciones no comestibles de verduras. (a) Extracto crudo (b) Extracto concentrado (c) Extracto deshidratado (polvo)
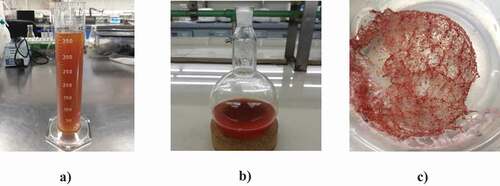
The determination of the (poly)-phenol content in the extracts and powder, based on the fresh weight of the residues (FW), allows to quantify the loss of both polyphenolic compounds and the antioxidant capacity during each concentration stage. The total (poly)-phenolic content in the crude extract, concentrated extract and powder, based on the IFV weight (fresh weight), were 93.6 ± 5.6, 75.7 ± 7.4, and 67.2 ± 2.2 (mg GAE/100 g FW), respectively. Likewise, the respective values of the antioxidant capacities, in the same order, were 49.0 ± 6.3, 40.3 ± 5.2, and 39.3 ± 1.7 (mg Fe2+/100 g FW). The results above indicate that the losses of (poly)-phenolic compounds are less than 20% in each stage, while during the vacuum evaporation at 55°C a greater loss occurs. It should be noted that, although freeze-drying is an appropriate technology for thermally labile compounds, the processing times and unit cost are significantly high. Therefore, its application is only justified to obtain products with very high added value. In order to produce the dehydrated extract of (poly)-phenolic compounds at higher productive scales, the spray-drying process is a possibility, a technology that in comparative terms is faster and cheaper. However, the operating conditions must be optimized to avoid accelerated degradation of the product of interest.
Nonetheless, the freeze-drying process showed a low impact in the stability of (poly)-phenolic compounds, results that are consistent with those published by Koffi et al. (Citation2015) for leaves of T. grandis. The foregoing shows that it is feasible to obtain a product with comparatively high values of (poly)-phenolic content and antioxidant capacity from IFV mixtures, and that the process conditions had moderate negative effects on the recovery yield of the compounds of interest and their properties.
4. Conclusions
It was technically feasible to recover (poly)-phenolic antioxidants from the mixture of inedible fractions of vegetables. The leaves and stems of the vegetables like broccoli, celery, carrots, beetroots, cauliflower, and cabbage presented a total (poly)-phenolic content of 267–32.71 mg GAE/100 g FW and antioxidant capacity of 176–13.6 mg Fe2+/100 g FW. The extraction conditions determined on the basis of experimental design results and the cost-benefit criteria were stirring speed of 100 rpm, temperature of 55°C, 60 min of extraction time, solid/liquid ratio of 1 g/5 mL, pH of 3, and a 20% ethanol/water solvent, which allowed to obtain an extract with (poly)-phenolic antioxidants from mixtures of the vegetables. A powder was successfully obtained whose (poly)-phenol concentration factor was approx. 20, with a TPC of 1758 ± 58 mg GAE/100 g and AOC of 1020 ± 44 mg Fe+2/100 g of dry powder. These results represent a progress toward the use of the mixture of inedible vegetable wastes. This powder-type product is an attractive source of natural antioxidants with the potential to be used in food or as functional ingredients. Consequently, it is important in future studies to evaluate this process on a pilot scale.
Disclosure statement
No potential conflict of interest was reported by the authors.
Additional information
Funding
References
- Ahmed, M. I., Xu, X., Sulieman, A. A., Mahdi, A. A., & Na, Y. (2020). Effect of extraction conditions on phenolic compounds and antioxidant properties of koreeb (Dactyloctenium aegyptium) seeds flour. Journal of Food Measurement and Characterization, 14(2), 799–808. https://doi.org/https://doi.org/10.1007/s11694-019-00328-9
- Aires, A., Carvalho, R., & Saavedra, M. J. (2017). Reuse potential of vegetable wastes (broccoli, green bean and tomato) for the recovery of antioxidant phenolic acids and flavonoids. International Journal of Food Science & Technology, 52(1), 98–107. https://doi.org/https://doi.org/10.1111/ijfs.13256
- Ajila, C. M., Aalami, M., Leelavathi, K., & Rao, U. J. S. P. (2010). Mango peels powder: A potential source of antioxidant and dietary fiber in macaroni preparations. Innovative Food Science & Emerging Technologies, 11(1), 219–224. https://doi.org/https://doi.org/10.1016/j.ifset.2009.10.004
- Alirezalu, K., Pateiro, M., Yaghoubi, M., Alirezalu, A., Peighambardoust, S. H., & Lorenzo, J. M. (2020). Phytochemical constituents, advanced extraction technologies and techno-functional properties of selected Mediterranean plants for use in meat products. A comprehensive review. Trends in Food Science & Technology, 100, 292–306. https://doi.org/https://doi.org/10.1016/j.tifs.2020.04.010
- Alves, E. M. H., Fonseca, A. P. R., Abreu, W. C., & Cardoso, A. P. M. (2017). Phenolic compounds and antioxidant activity of tuberous root leaves. International Journal of Food Properties, 20(12), 2966–2973. https://doi.org/https://doi.org/10.1080/10942912.2016.1263654
- Angulo, J., Mahecha, L., Yepes, S., Yepes, A. M., Bustamante, G., Jaramillo, H., Valencia, E., Villamil, T., & Gallo, J. (2012). Nutritional evaluation of fruit and vegetable waste as feedstuff for diets of lactating Holstein cows. Journal of Environmental Management, 95, S210–S214. https://doi.org/https://doi.org/10.1016/j.jenvman.2011.06.050
- AOAC. (2006). Official methods of analysis (18th ed., (W.Horwitz, Ed.)). Association of Official AnalyticalChemists.
- Apak, R., Özyürek, M., Güclü, K., & Çapanoğlu, E. (2016). Antioxidant Activity/Capacity measurement. 1. Classification, physicochemical principles, mechanisms, and electron transfer (ET)-based assays. Journal of Agricultural and Food Chemistry, 64(5), 997–1027. https://doi.org/https://doi.org/10.1021/acs.jafc.5b04739
- Babbar, N., Oberoi, H. S., Sandhu, S. K., & Bhargav, V. K. (2014). Influence of different solvents in extraction of phenolic compounds from vegetable residues and their evaluation as natural sources of antioxidants. Journal of Food Science and Technology, 51(10), 2568–2575. https://doi.org/https://doi.org/10.1007/s13197-012-0754-4
- Barrett, D. M., Beaulieu, J. C., & Shewfelt, R. (2010). Color, flavor, texture, and nutritional quality of fresh-cut fruits and vegetables: Desirable levels, instrumental and sensory measurement, and the effects of processing. Critical Reviews in Food Science and Nutrition, 50(5), 369–389. https://doi.org/https://doi.org/10.1080/10408391003626322
- Bengardino, M. B., Fernández, M. V., Nutter, J., Jagus, R. J., & Agüero, M. V. (2019). Recovery of bioactive compounds from beet leaves through simultaneous extraction: Modelling and process optimization. Food and Bioproducts Processing, 118, 227–236. https://doi.org/https://doi.org/10.1016/j.fbp.2019.09.013
- Benzie, I. F., & Strain, J. J. (1996). The ferric reducing ability of plasma (FRAP) as a measure of “antioxidant power”: The FRAP assay. Analytical Biochemistry, 239(1), 70–76. https://doi.org/https://doi.org/10.1006/abio.1996.0292
- Biondo, P. B. F., Boeing, J. S., Barizão, E. O., De Souza, N. E., Matsushita, M., Oliveira, C. C., Boroski, M., & Visentainer, J. V. (2014). Evaluation of beetroot (Beta vulgaris L.) leaves during its developmental stages: A chemical composition study. Food Science and Technology, 34(1), 94–101. https://doi.org/https://doi.org/10.1590/S0101-20612014005000007
- Blasi, F., & Cossignani, L. (2020). An overview of natural extracts with antioxidant activity for the improvement of the oxidative stability and shelf life of edible oils. Processes, 8(8), 956. https://doi.org/https://doi.org/10.3390/pr8080956
- Blois, M. S. (1958). Antioxidant determination by use of a stable free radical. Nature, 181(4617), 1199–1200. https://doi.org/https://doi.org/10.1038/1811199a0
- Bucic-Kojic, A., Planinic, M., Srecko, T., Bilic, M., & Velic, D. (2007). Study of solid-liquid extraction kinetics of total polyphenols from grape seeds. Journal of Food Engineering, 81(1), 236–242. https://doi.org/https://doi.org/10.1016/j.jfoodeng.2006.10.027
- Burri, S. C., Ekholm, A., Håkansson, Å., Tornberg, E., & Rumpunen, K. (2017). Antioxidant capacity and major phenol compounds of horticultural plant materials not usually used. Journal of Functional Foods, 38, 119–127. https://doi.org/https://doi.org/10.1016/j.jff.2017.09.003
- Cartea, M. E., Francisco, M., Soengas, P., & Velasco, P. (2011). Phenolic compounds in Brassica vegetables. Molecules, 16(1), 251–280. https://doi.org/https://doi.org/10.3390/molecules16010251
- Cimmino, R., Barone, C. M. A., Claps, S., Varricchio, E., Rufrano, D., Caroprese, M., Albenzio, M., De Palo, P., Campanile, G., & Neglia, G. (2018). Effects of dietary supplementation with polyphenols on meat quality in Saanen goat kids. Veterinary Research, 14(1), 181. https://doi.org/https://doi.org/10.1186/s12917-018-1513-1
- Cuevas-Valenzuela, J., González-Rojas, Á., Wisniak, J., Apelblat, A., & Pérez-Correa, J. R. (2014). Solubility of (+)-catechin in water and water-ethanol mixtures within the temperature range 277.6-331.2 K: Fundamental data to design polyphenol extraction processes. Fluid Phase Equilibria, 382, 279–285. https://doi.org/https://doi.org/10.1016/j.fluid.2014.09.013
- Cujic, N., Savikin, K., Jankovic, T., Pljevljakusic, D., Zdunic, G., & Ibric, S. (2016). Optimization of polyphenols extraction from dried chokeberry using maceration as traditional technique. Food Chemistry, 194, 135–142. https://doi.org/https://doi.org/10.1016/j.foodchem.2015.08.008
- D’Alessandro, L. G., Kriaa, K., Nikov, I., & Dimitrov, K. (2012). Ultrasound assisted extraction of polyphenols from black chokeberry. Separation and Purification Technology, 93, 42–47. https://doi.org/https://doi.org/10.1016/j.seppur.2012.03.024
- Devkota, A. R., Dhakal, D. D., Gautam, D. M., & Dutta, J. P. (2014). Assessment of fruit and vegetable waste at wholesale markets in Nepal for vermicomposting. International Journal of Research, 1, 1–9.
- Domínguez-Perles, R., Martínez-Ballesta, M. C., Carvajal, M., García-Viguera, C., & Moreno, D. A. (2010). Broccoli-derived by-products-A promising source of bioactive ingredients. Journal of Food Science, 75(4), C383–C392. https://doi.org/https://doi.org/10.1111/j.1750-3841.2010.01606.x
- Feduraev, P., Chupakhina, G., Maslennikov, P., Tacenko, N., & Skrypnik, L. (2019). Variation in phenolic compounds content and antioxidant activity of different plant organs from Rumex crispus L. and Rumex obtusifolius L. at different growth stages. Antioxidants, 8(7), 237. https://doi.org/https://doi.org/10.3390/antiox8070237
- Fierascu, R. C., Fierascu, I., Avramescu, S. M., & Sieniawska, E. (2019). Recovery of natural antioxidants from agro-industrial side streams through advanced extraction techniques. Molecules, 24(23), 4212. https://doi.org/https://doi.org/10.3390/molecules24234212
- Fukumoto, L. R., & Mazza, G. (2000). Assessing antioxidant and prooxidant activities of phenolic compounds†. Journal of Agricultural and Food Chemistry, 48(8), 3597–3604. https://doi.org/https://doi.org/10.1021/jf000220w
- Gonzales, G. B., Raes, K., Coelus, S., Struijs, K., Smagghe, G., & Van Camp, J. (2014). Ultra (high)-pressure liquid chromatography–electrospray ionization-time-of-flight-ion mobility-high definition mass spectrometry for the rapid identification and structural characterization of flavonoid glycosides from cauliflower waste. Journal of Chromatography A, 1323, 39–48. https://doi.org/https://doi.org/10.1016/j.chroma.2013.10.077
- Goyeneche, R., Di Scala, K., Ramirez, C. L., & Fanovich, M. A. (2020). Recovery of bioactive compounds from beetroot leaves by supercritical CO2 extraction as a promising bioresource. The Journal of Supercritical Fluids, 155, 104658. https://doi.org/https://doi.org/10.1016/j.supflu.2019.104658
- Huang, J.-H., & Lim, S.-B. (2015). Antioxidant and anticancer activities of broccoli by-products from different cultivars and maturity stages at harvest. Preventive Nutrition and Food Science, 20(1), 8. https://doi.org/http://dx.doi.org/10.3746/pnf.2015.20.1.8
- Jafarzadeh, S., Jafari, S. M., Salehabadi, A., Nafchi, A. M., Kumar, U. S. U., & Khalil, H. P. S. A. (2020). Biodegradable green packaging with antimicrobial functions based on the bioactive compounds from tropical plants and their by-products. Trends in Food Science & Technology, 100, 262–277. https://doi.org/https://doi.org/10.1016/j.tifs.2020.04.017
- Khedkar, M. A., Nimbalkar, P. R., Chavan, P. V., Chendake, Y. J., & Bankar, S. B. (2017). Cauliflower waste utilization for sustainable biobutanol production: Revelation of drying kinetics and bioprocess development. Bioprocess and Biosystems Engineering, 40(10), 1493–1506. https://doi.org/https://doi.org/10.1007/s00449-017-1806-y
- Koffi, E. N., Meudec, E., Adjé, F. A., Lozano, P. R., Lozano, Y. F., & Bekro, Y. A. (2015). Effect of reverse osmosis concentration coupled with drying processes on polyphenols and antioxidant activity obtained from Tectona grandis leaf aqueous extracts. Journal of Applied Research on Medicinal and Aromatic Plants, 2(2), 54–59. https://doi.org/https://doi.org/10.1016/j.jarmap.2015.03.001
- Kooti, W., & Daraei, N. (2017). A review of the antioxidant activity of celery (Apium graveolens L). Journal of Evidence-based Complementary & Alternative Medicine, 22(4), 1029–1034. https://doi.org/https://doi.org/10.1177/2156587217717415
- Leite, C. W., Boroski, M., Boeing, J. S., Aguiar, A. C., França, P. B., De Souza, N. E., & Visentainer, J. V. (2011). Chemical characterization of leaves of organically grown carrot Dacus carota L.) in various stages of development for use as food. Food Science and Technology, 31(3), 735–738. https://doi.org/http://dx.doi.org/10.1590/S0101-20612011000300028
- Liang, Y., Li, Y., Zhang, L., & Liu, X. (2019). Phytochemicals and antioxidant activity in four varieties of head cabbages commonly consumed in China. Food Production, Processing and Nutrition, 1(1), 3. https://doi.org/https://doi.org/10.1186/s43014-019-0003-6
- Llorach, R., Tomás-Barberán, F. A., & Ferreres, F. (2004). Lettuce and chicory byproducts as a source of antioxidant phenolic extracts. Journal Agricultural Food Chemistry, 52(16), 5109–5116. https://doi.org/https://doi.org/10.1021/jf040055a
- Majerska, J., Michalsk, A., & Figiel, A. (2019). A review of new directions in managing fruit and vegetable processing by-products. Trends Food Science & Technology, 88, 207–219. https://doi.org/https://doi.org/10.1016/j.tifs.2019.03.021
- Miranda, S. (2018). La recuperación alimentaria en Lo Valledor, producto de una prometedora estrategia en gestión de residuos. In D. Acuña, A. Domper, P. Eguillor, C. G. González, & I. Zacarías. (Eds.), Manual de pérdidas y desperdicios de alimentos. 5 Al Día Chile – INTA Universidad de Chile – Ministerio de Agricultura (pp. 95–102). A IMPRESORES. http://fucoa.cl/manual_residuos/
- Nasrin, T. A. A., & Matin, M. A. (2018). Valorization of vegetable wastes. In A. K. Anal (Ed..), Food processing by-products and their utilization (pp. 53–80). John Wiley & Sons Ltda. Published.
- Ninfali, P., & Angelino, D. (2013). Nutritional and functional potential of Beta vulgaris cicla and rubra. Fitoterapia, 89, 188–199. https://doi.org/https://doi.org/10.1016/j.fitote.2013.06.004
- Pacifico, S., Piccolella, S., & Veneziano, R. (2019, April 3–5). New sustainable cosmetic products from food waste: A joined-up approach between design and food chemistry. In Designing sustainability for all, proceedings of the 3rd LeNS world distributed conference (3rd volume). Ambrosio, M., Vezzoli, C., Eds.; Edizioni POLI.design: Milano, Italy, 2019; pp. 975–979. http://lensconference3.org/index.php/program/presentations/item/144-new-sustainable-cosmetic-products-from-food-waste-a-joined-up-approach-between-design-and-food-chemistry
- Pang, S. F., Yusoff, M. M., & Gimbun, J. (2014). Extraction and microencapsulation of polyphenols from Orthosiphon stamineus leaves. Journal of Mechanical Engineering and Sciences, 7(1), 1033–1041. https://doi.org/http://dx.doi.org/10.15282/jmes.7.2014.2.0100
- Pradal, D., Vauchel, P., Decossin, S., Dhulster, P., & Dimitrov, K. (2016). Kinetics of ultrasound-assisted extraction of antioxidant polyphenols from food by-products: Extraction and energy consumption optimization. Ultrasonics Sonochemistry, 32, 137–146. https://doi.org/https://doi.org/10.1016/j.ultsonch.2016.03.001
- Punzi, R., Paradiso, A., Fasciano, C., Trani, A., Faccia, M., De Pinto, M. C., & Gambacorta, G. (2014). Phenols and antioxidant activity in vitro and in vivo of aqueous extracts obtained by ultrasound-assisted extraction from Artichoke by-products. Natural Product Communications, 9(9), 1315–1318. https://doi.org/https://doi.org/10.1177/1934578X1400900924
- Roytrakul, S., & Verpoorte, R. (2007). Role of vacuolar transporter proteins in plant secondary metabolism: Catharanthus roseus cell culture. Phytochemistry Reviews, 6(2–3), 383–396. https://doi.org/https://doi.org/10.1007/s11101-006-9022-4
- Sagar, N. A., Pareek, S., Sharma, S., Yahia, E. M., & Lobo, M. G. (2018). Fruit and vegetable waste: Bioactive compounds, their extraction, and possible utilization. Comprehensive Reviews in Food Science and Food Safety, 17(3), 512–531. https://doi.org/https://doi.org/10.1111/1541-4337.12330
- Saini, A., Panesar, P. S., & Bera, M. B. (2019). Valorization of fruits and vegetables waste through green extraction of bioactive compounds and their nanoemulsions-based delivery system. Bioresources and Bioprocessing, 6(1), Article 26. https://doi.org/https://doi.org/10.1186/s40643-019-0261-9
- Santos, M. C. P., Mendes, N. S., Cameron, L. C., Ferreira, M. L. S., & Gonçalves, E. C. B. A. (2020). Optimization of chemical extraction of phenolic compounds from fruits and vegetable residue by UPLC-MSE. International Journal of Food Properties, 23(1), 2144–2159. https://doi.org/https://doi.org/10.1080/10942912.2020.1843485
- Shi, J., Yu, J., Pohorly, J., Young, J., Bryan, M., & Wu, Y. (2003). Optimization of the extraction of polyphenols from grape seed meal by aqueous ethanol solution. Journal of Food, Agriculture & Environment, 1(2), 42–47.
- Singleton, V. L., Orthofer, R., & Lamuela-Raventós, R. M. (1999). Analysis of total phenols and other oxidation substrates and antioxidants by means of Folin-Ciocalteu reagent. In L. Packer (Ed.), Methods in enzymology (Vol. 299, pp. 152–178). Academic press. https://doi.org/https://doi.org/10.1016/S0076-6879(99)99017-1
- Wang, S., Meckling, K. A., Marcone, M. F., Kakuda, Y., & Tsao, R. (2011). Synergistic, additive, and antagonistic effects of food mixtures on total antioxidant capacities. Journal of Agricultural and Food Chemistry, 59(3), 960–968. https://doi.org/https://doi.org/10.1021/jf1040977
- Yao, Y., & Ren, G. (2011). Effect of thermal treatment on phenolic composition and antioxidant activities of two celery cultivars. LWT-Food Science and Technology, 44(1), 181–185. https://doi.org/https://doi.org/10.1016/j.lwt.2010.07.001