ABSTRACT
The relative available energy (RAE) based on the fermentability of breath hydrogen excreted by gut microbiota was assessed using a method accepted by the Japanese Society for Dietary Fiber Research. The purpose of this study was to evaluate RAE of difructose anhydride III (DFA III) and maltobionic acid (MA). In this study, which had a within-subject, repeated measures design, 11 healthy female participants ingested 5 g of DFA III, MA, and fructooligosaccharide, which has RAE of 2 kcal/g. Breath hydrogen was collected 12 times up to 14 h after meal consumption and measured using gas chromatography, and the area under the curves (AUCs) was calculated 0–14 h after ingestion. Compared with the AUCs of breath hydrogen excretion related to the ingested fructooligosaccharide for 14 h, those of DFA III and MA were 40.5% and 23.1%, respectively. RAE was 0.81 kcal/g for DFA III and 0.46 kcal/g for MA, respectively.
RESUMEN
El presente estudio se propuso evaluar la energía relativa disponible (RAE) a partir de la fermentabilidad del hidrógeno contenido en el aliento producido por la microbiota intestinal, utilizando para ello un método aceptado por la Sociedad Japonesa para la Investigación de la Fibra Dietética. El objetivo del estudio fue evaluar la RAE del anhídrido de difructosa III (DFA III) y del ácido maltobiónico (MA). Así, empleando un diseño de medidas repetidas en un mismo sujeto, 11 mujeres sanas ingirieron cinco gramos de DFA III, MA y fructooligosacárido, cantidad que posee un RAE de 2 kcal/g. Se recogió hidrógeno del aliento 12 veces hasta 14 horas después del consumo de alimentos, midiéndolo mediante cromatografía de gases y calculando el área bajo las curvas (AUC) de 0 a 14 horas después de la ingestión. En comparación con las AUC de la excreción de hidrógeno en el aliento relacionadas con el fructooligosacárido ingerido durante 14 horas, las de DFA III y MA fueron 40.5% y 23.1%, respectivamente, mientras que el RAE fue de 0.81 kcal/g para el DFA III y de 0.46 kcal/g para el MA, respectivamente.
1. Introduction
The energy value of a food item is essential when creating nutritional labeling for processed food and nutrition education. The energy value of food that is digested and/or absorbed in the upper gastrointestinal tract should be determined. However, the available energy values of indigestible or nondigestible carbohydrates such as dietary fibres, resistant starch, nondigestible oligosaccharides, and sugar alcohols change following fermentation in the lower gastrointestinal tract. Livesey reported that the available energy of digestible carbohydrates is 4 kcal/g and that of fully fermentable carbohydrates is 2 kcal/g (Livesey, Citation2001). This energy value has been derived from several proposed equations (Livesey, Citation2001; Oku, Citation1996; Roberfroid, Citation1999). In the European Union, USA, Canada, and Australia/New Zealand, the energy value of fermentable fibres is also adopted at 2 kcal/g (Bureau of Nutritional Sciences, Food Directotrate, Health Products and Food Branch, Health Canada, Citation2012). In standard tables of food composition in Japan in 2020, the energy of carbohydrates that are not digested in the small intestine and are completely fermented in the large intestine is 2 kcal/g, which is the same as that established in other countries (The Council for Science and Technology Ministry of Education, Culture, Sports, Science and Technology, Japan, Citation2020). However, nondigestible carbohydrates are not fermented by intestinal microbes, so their available energy is 0 kcal/g. Therefore, the available energy of nondigestible fermentable carbohydrates is 0–2 kcal/g. In Japan, the Japanese Society for Dietary Fiber Research is taking the lead in evaluating the available energy of new nondigestible carbohydrate materials on an individual basis.
Nondigestible carbohydrates as dietary fibres are partially or completely fermented by gut microbiota and metabolized into short-chain fatty acids (SCFAs), carbon dioxide, hydrogen, methane, amino acid, and other compounds. SCFAs are absorbed in the large intestine and used solely as an energy source by the host. Therefore, the available energy of nondigestible and fermentable carbohydrates is dependent on the amount of SCFAs produced in the fermentation process by gut microbiota. The fermentability of the test material must be assessed accurately as it can be influenced by the amount of SCFAs produced, although it can be challenging in case of humans. Therefore, breath hydrogen excretion has been measured as an indicator of fermentation because hydrogen cannot be produced by humans but only by gut microbiota (Livesey et al., Citation1993; Rumessen, Citation1992; Storey et al., Citation1998). Recently, Oku et al. evaluated the available energy of newly developed carbohydrate food materials using an indirect and simple method based on the fermentability of hydrogen excreted by breath (Nakamura & Oku, Citation2005; Oku & Nakamura, Citation2014). The Japanese Society for Dietary Fiber Research has accepted this method of evaluation, and the available energy of dietary fibre, resistant starch, resistant maltodextrin, resistant glucan, nondigestible oligosaccharide, sugar alcohol, and rare sugar has been evaluated using fructooligosaccharide (FOS) as a reference (Hamanaka et al., Citation2020; Nakamura et al., Citation2020, Citation2017; Oku & Nakamura, Citation2002, Citation2014). When assessing the fermentability of nondigestible saccharides, we recommend observation of breath hydrogen excretion for a minimum of 14 h after ingestion of the test material due to the substantial variation of the degree of fermentation undergone by the nondigestible carbohydrate (Oku & Nakamura, Citation2014).
In a previous study that assessed the available energy of difructose anhydride III (DFA III) and maltobionic acid (MA), the fermentability of DFA III and MA was evaluated by assessing the exhaled breath gas 8 h after ingestion, although it might have been done with some inaccuracy. In the present study, we evaluated the available energy of two carbohydrate food materials, DFA III and MA, using an indirect and simple method based on the fermentability of breath hydrogen excretion for more than 14 h.
2. Materials and methods
2.1. Ethical approval
All experiments were compliant with the code of ethics of the World Medical Association (Declaration of Helsinki, October 2013). The study protocol involving humans was approved by the Ethical Committee Concerning Research in Humans of Nagoya Women’s University (approval nos. 29-4 and 29-15, Nagoya, Japan). All participants were asked to provide written informed consent to participate in the study. All experiments were conducted in the laboratory of Public Health Nutrition at Nagoya Women’s University.
2.2. Test materials
DFA III powder (>99.9% purity; Nippon Beet Sugar Manufacturing Co., Ltd., Obihiro, Japan) is a cyclically linked disaccharide that consists of two moles of fructose and stimulates the intestinal absorption of calcium and other minerals. MA syrup (>98.0% purity as solid; San-ei Sucrochemical Co., Ltd., Aichi, Japan), which is composed of a gluconic acid and a glucose linked by an α-1,4 bond, is highly resistant to digestion and fermentation and contributes to improved flavor of various food and juices as well as mineral solubilization (Fukami et al., Citation2019; Suehiro et al., Citation2019). FOS powder (>98.0% purity, Meiji Co., Ltd., Tokyo, Japan) was used as a reference material. FOS is a typical nondigestible oligosaccharide that cannot be hydrolyzed by digestive enzymes and is fermented completely by gut microbiota. It is composed of 1-kestose (36.8%), nystose (51.9%), and 1F-β-fructofranosyl-nystose (9.3%), and the remaining 1% is comprised of glucose and sucrose.
2.3. Participants
Eleven healthy female participants voluntarily participated in this study. Their mean age was 21.6 ± 1.2 years, height was 158.5 ± 4.7 cm, body weight was 53.0 ± 6.8 kg, and body mass index (BMI) was 21.1 ± 2.7 kg/m2. The exclusion criteria included a history of gastrointestinal diseases, carbohydrate malabsorption, diabetes, pulmonary diseases, or BMI ≥25.0 kg/m2. Before the experiment, it was confirmed that all participants were hydrogen producers and not methane producers. The participants had not taken any antibiotics or laxatives in the 2 weeks prior to the experiments.
2.4. Experimental protocol
All test materials were administered in random order at intervals of ≥1 week during the experimental period. Experiments were conducted under the direction of a physician. The experiment was performed using the methods employed in our previous studies (Kitagawa et al., Citation2018; Nakamura et al., Citation2016; Oku et al., Citation2005; Tachibe et al., Citation2011; Tagami et al., Citation2012; Tanabe et al., Citation2018), and the experimental protocol is shown in . On the day before the ingestion of the test material, the participants were asked to finish their evening meal until 9 p.m. and were only allowed to drink water from 10 p.m. onwards. The participants arrived at our laboratory on the morning of the day of the experiment, where their health status was examined and their blood pressure and pulse rate were measured. Then, 750 mL of end-expiratory gas was collected using a collection bag (Quin Tron Instrument, WI, USA). After ingestion of the test material, end-expiratory gas was collected at 1-h intervals for 8 h and at 2-h intervals until 14 h to measure breath hydrogen.
For the test, the participants ingested the equivalent of 5 g of FOS, DFA III, or MA dissolved in 150 mL of distilled water. The amount of ingested test material did not induce transitory osmotic diarrhea. The breath hydrogen concentration was measured using a BGA-1000D Breath Gas Analyzer (Laboratory for Expiration Biochemistry Nourishment Metabolism Co., Ltd., Nara, Japan).
The participants were prohibited from ingesting foods containing nondigestible carbohydrates for 3 days before each experimental day. During the experiment, they were also prohibited from ingesting foods or beverages, except warm water and tea, as well as from sleeping and smoking. The participants were required to sit on a chair and were prohibited from exercising with hyperventilation until the final collection of end-respiratory gas.
2.5. Exprrimental meals for the experiment
Food intake was restricted for the participants during the experimental period. The experimental meals, from which hydrogen was neither produced nor detected, were given to the participants at 3, 6, 9, and 12 h after ingestion of the test material. shows the nutritional composition of the experimental meals ingested by the participants during an experiment. Canned tuna fish (Sea-chicken mild, 80 g; Hagoromo Food, Shizuoka, Japan), boiled egg (50 g), boiled chicken (Salad chicken, 58 g; Prima Meat Packers, Ltd, Tokyo, Japan), and tea containing 10 g of table sugar were the components of the experimental meals. The energy supplied to the participants on an experimental day was 932 kcal with 76 g of protein (). Participants who were particularly hungry when consuming the experimental meals were given additional sugar and boiled eggs. In addition, each participant was given a multivitamin tablet containing minerals (Nature Made, Otsuka Pharmaceutical, Tokyo, Japan) as a supplement during the experimental period when the participant placed a request.
Table 1. Nutrition composition of the experimental meals ingested by the participants during an experiment.
Tabla 1. Composición nutricional de los alimentos experimentales ingeridos por las participantes durante el experimento
Table 2. Total nutritional components of the experimental meals ingested by the participants during an experiment.
Tabla 2. Componentes nutricionales totales de los alimentos experimentales ingeridos por las participantes durante el experimento
2.6. Evaluation of the relative available energy of difructose anhydride III and maltobionic acid
The available energy of DFA III and MA was calculated based on the area under the curve (AUC) of the breath hydrogen concentration of FOS, which has 2 kcal/g of available energy (Oku et al., Citation2009).
2.7. Statistical analysis
Mean and standard deviation (SD) values were calculated for breath hydrogen concentration at each specified time point after ingestion of the test material. AUC analyses were performed for breath hydrogen concentration. Following the verification of a normal or non-normal distribution, one-way analysis of variance and Tukey HSD test were conducted. Statistical analysis was performed using SPSS version 24 (SPSS Inc., Japan), and statistical significance was set at p < .05.
3. Results and discussion
The participants neither dropped out of the study nor experienced side effects. They maintained a healthy status throughout the study period. The average energy intake per participant was 932 kcal/day, with a protein intake of 76 g/day. To estimate the available energy, 5 g of DFA III, MA, or FOS were ingested by the participants. shows time-dependent changes in breath hydrogen excretion after ingestion of the test materials up to 14 h. shows the AUC of breath hydrogen excretion after ingestion of the test materials up to 14 h.
Figure 2. Time-dependent changes in breath hydrogen excretion after ingestion of the test materials.
Figura 2. Cambios dependientes del tiempo en la excreción de hidrógeno por el aliento tras la ingestión de los materiales de prueba
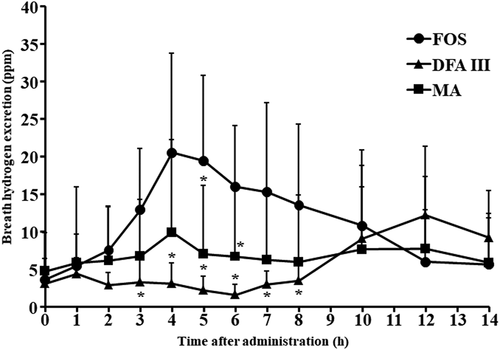
Figure 3. Area under the curve of breath hydrogen excretion after ingestion of the test materials.
Figura 3. Área bajo la curva de excreción de hidrógeno por el aliento tras la ingestión de los materiales de ensayo
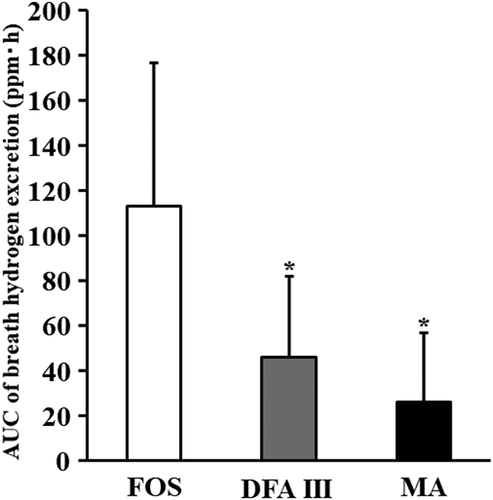
3.1. Difructose anhydride III
DFA III is a nondigestible disaccharide with a resistance for digestion and absorption in the small intestine (Kikuchi et al., Citation2004). Almost no breath hydrogen excretion occurs for 8 h after ingestion of DFA III (Tamura et al., Citation2003). After ingestion of DFA III, breath hydrogen excretion was not different from fasting up to 8 h after ingestion, but it increased slowly thereafter, peaking at 12 h after ingestion (12.2 ± 9.2 ppm). There were significant differences at 3, 4, 5, 6, 7 and 8 h compared with those in the FOS group (p < .05). A comparison of breath hydrogen excretion showed no significant difference between 12 and 14 h. Up to 14 h, the identified AUC of DFA III (45.8 ± 35.9 ppm for 14 h) was significantly lower than that of FOS (111.7 ± 59.7 ppm for 14 h) (p < .05). On comparing the AUC of DFA III between 8 h (5.4 ± 4.0 ppm for 14 h) and 14 h, the AUC for 14 h was nine times higher than that for 8 h (p < .05). After the ingestion of DFA III, almost no breath hydrogen excretion was observed up to 8 h and breath hydrogen excretion started after 8 h, which confirmed that DFA III was a slowly fermentable oligosaccharide. DFA III starts excreting hydrogen 8 h after ingestion and follows the same fermentation pattern as high-molecular-weight dietary fibre (Oku & Nakamura, Citation2014). The dynamics of breath hydrogen excretion up to 8 h after DFA III ingestion observed in the present study were in agreement with the findings of Tamura et al. (Citation2003, Citation2004). These reports showed that DFA III ferments slowly and is poorly fermentable, distinguishing it from highly fermentable FOS and other fermentable nondigestible oligosaccharides.
3.2. Maltobionic acid
Breath hydrogen excretion after intake of MA increased slightly after 4 h (9.9 ± 12.3 ppm) but was not significantly different from that at fasting. When the AUC of MA was compared with that of FOS, MA was significantly lower up to 14 h (26.1 ± 30.7 ppm for 14 h, p < .05). In an in vitro digestibility study using mucosal enzymes from the rat small intestine, the digestibility of MA was found to be equivalent to that of maltitol, suggesting that MA is a nondigestible saccharide (Fukami et al., Citation2020). The study also revealed that MA was less utilized by gut microbiota than maltitol. We previously reported that almost no breath hydrogen excretion occurs for 8 h after MA ingestion (Tanabe et al., Citation2020), which might indicate that MA is a poorly fermentable and nondigestible oligosaccharide.
Compared with the AUC of breath hydrogen excretion after the ingestion of MA for up to 14 h and 8 h, those related to 14 h were twice higher than those related to 8 h. These results show that MA was gradually fermented by the gut microbiota but was a poorly fermented oligosaccharide compared with DFA III and FOS. Moreover, we also reported the dynamics in the gastrointestinal tract concerning digestion, absorption, and fermentation in the participant following MA ingestion for 8 h (Tanabe et al., Citation2020). Postprandial glucose, insulin, and breath hydrogen excretion concentrations following MA ingestion showed almost no change. Based on these findings, MA may be considered a poorly fermentable and nondigestible oligosaccharide.
3.3. Fructooligosaccharide
Following the ingestion of FOS, breath hydrogen excretion was marginally increased and showed a peak (20.5 ± 13.2 ppm) after 4 h. Our previous studies showed that the excretion of breath hydrogen reaches a peak at 4–6 h after ingestion of 5 g of FOS in humans, and the results of the present study support our previous finding (Oku & Nakamura, Citation2014; Tanabe et al., Citation2020). Subsequently, breath hydrogen excretion moderately declined and returned to the fasting level (5.6 ± 6.1 ppm) after 14 h of ingestion. It can be assumed that almost all FOS is fermented at approximately 14 h after the ingestion of 5 g of FOS. In a previous report, we found that the AUC of breath hydrogen excretion was not significantly different between 14 h and 24 h after ingestion (Oku & Nakamura, Citation2014). This result showed that when measuring the fermentability of easily fermentable nondigestible oligosaccharides in terms of breath hydrogen excretion, exhaled gas must be collected for at least 14 h.
3.4. Evaluation trial of the available energy from difructose anhydride III and maltibionic acid
We expressed the energy value derived from using the AUCs of breath hydrogen excretion as the relative ratio to FOS (Oku & Nakamura, Citation2014). According to the standard tables of food composition in Japan in 2020, FOS is classified as a nondigestible oligosaccharide with an energy value of 2 kcal/g. show the results obtained after assessing the fermentability and energy values of DFA III and MA based on the AUC of breath hydrogen excretion in comparison with FOS at 8 and 14 h after ingestion. The energy was calculated under the assumption that all three saccharides reached the large intestine after undergoing almost no hydrolysis in the small intestine. Compared with the results obtained from the AUC of breath hydrogen excretion for 14 h after FOS ingestion, the relative available energy was 0.81 kcal (2 kcal/g × 40.5%/100% = 0.81 kcal/g) and 0.46 kcal/g (2 kcal/g × 23.1%/100% = 0.46 kcal/g) for MA. Because numerical values containing two decimal places are not practical for usage in nutrition education and nutritional labeling, the nearest whole numbers were considered. Therefore, the relative available energy values were 1 kcal/g for DFA III and 0 kcal/g for MA. However, when the relative available energy values were evaluated based on the AUC of breath hydrogen excretion, the relative energy for 8 h was 0.14 kcal/g (2 kcal/g × 6.9%/100% = 0.14 kcal/g) for DFA III and 0.35 kcal/g (2 kcal/g × 17.6%/100% = 0.35 kcal/g) for MA. In case of 8 h collection of breath hydrogen, the relative available energy was 0 kcal/g for both DFA III and MA. These results showed that a breath collection period of 8 h cannot be used for accurate evaluation of the fermentability of nondigestible oligosaccharides. Therefore, experiments assessing fermentability should end within 24 h; thus, we considered 14 h as the maximum length of time for participants to be subjected to breath collection.
Table 3. Energy estimation from the ratio of areas under the curve versus fructooligosaccharide.
Tabla 3. Estimación de la energía a partir de la ratio de las áreas bajo la curva versus el fructooligosacárido
Acknowledgments
The authors thank Meiji (Tokyo, Japan) for providing FOS.
Disclosure statement
No potential conflict of interest was reported by the author(s).
Additional information
Funding
References
- Bureau of Nutritional Sciences, Food Directotrate, Health Products and Food Branch, Health Canada. (2012). Policy for labelling and advertising of dietary fibre-containing food products.
- The Council for Science and Technology Ministry of Education, Culture, Sports, Science and Technology, Japan. (2020). Standard tables of food composition in Japan – 2020 - Available carbohydrates, polyols, dietary fiber and organic acids [ Online]. Retrieved March 23, 2021, from https://www.mext.go.jp/content/20201225-mxt_kagsei-mext_01110_041.pdf
- Fukami, K., Suehiro, D., & Ohnishi, M. (2020). In vitro utilization characteristics of maltobionic acid and its effects on bowel movements in healthy humans. Journal of Applied Glycoscience, 67(1), 1–9. https://doi.org/https://doi.org/10.5458/jag.jag.JAG-2019_0013
- Fukami, K., Suehiro, D., & Takara, T. (2019). Safety evaluation of long-term intake and effects on bone mineral density of corn syrup solids containing maltobionic acid (maltobionic acid calcium salt) in healthy Japanese women. Japan Pharmacology Therapy, 47(2), 229–245. http://www.pieronline.jp/content/article/0386-3603/47020/229
- Hamanaka, M., Stewart, M., Miyahara, K., Nakamura, S., & Oku, T. (2020). Comparison of utilisation and fermentation of highly cross-linked phosphate starches produced from two different plant origins, potato and tapioca in rats and humans. International Journal of Food Sciences and Nutrition, 71(8), 1019–1031. https://doi.org/https://doi.org/10.1080/09637486.2020.1754349
- Kikuchi, H., Nagura, T., Inoue, M., Kishida, T., Sakurai, H., Yokota, A., Asano, K., Tomita, F., Sayama, K., & Senba, Y. (2004). Physical, chemical and physiological properties of difructose anhydride III produced from inulin by enzymatic reaction. Journal of Applied Glycoscience, 51(4), 291–296. https://doi.org/https://doi.org/10.5458/jag.51.291
- Kitagawa, M., Tanaka, M., Yoshikawa, Y., Iida, T., & Kishimoto, Y. (2018). Evaluation of absorption and fermentability of D-mannose, D-sorbose, and D-allose in humans. Japanese Association for Dietary Fiber Research, 22(2), 75–82. https://doi.org/https://doi.org/10.5458/jag.jag.JAG-2019_0010
- Livesey, G. (2001). A perspective on food energy standards for nutrition labelling. British Journal of Nutrition, 85(3), 271–287. https://doi.org/https://doi.org/10.1079/BJN2000253
- Livesey, G., Johnson, T., Gee, J. M., Smith, T., Lee, W. E., Hillan, K. A., Meyer, J., & Turner, S. C. (1993). Determination’ of sugar alcohol and polydextrose absorption in humans by the breath hydrogen (H2) technique: The stoichiometry of hydrogen production and the interaction between carbohydrates assessed in vivo and in vitro. European Journal of Clinical Nutrition, 47(6), 419–430.
- Nakamura, S., & Oku, T. (2005). Evaluation of available energy of several dietary fiber materials based on the fermentability from breath hydrogen excretion in healthy human subjects. Japanese Association for Dietary Fiber Research, 9(1), 34–46. https://doi.org/https://doi.org/10.11217/jjdf2004.9.34
- Nakamura, S., Tanabe, K., Morita, S., Hamaguchi, N., Shimura, F., & Oku, T. (2016). Metabolism and bioavailability of newly developed dietary fiber materials, resistant glucan and hydrogenated resistant glucan, in rats and humans. Nutrition & Metabolism, 13(1), 1–12. https://doi.org/https://doi.org/10.1186/s12986-016-0073-2
- Nakamura, S., Tanabe, K., Yamate, M., Osada, S., & Oku, T. (2020). Trial of available energy evaluation of highly cross-linked starch and modified cellulose based on breath H2 excretion. Current Nutrition & Food Science, 16(5), 794–801. https://doi.org/https://doi.org/10.2174/1573401315666190723145558
- Nakamura, S., Tanabe, K., Yoshinaga, K., Shimura, F., & Oku, T. (2017). Effects of 1,5-anhydroglucitol on postprandial blood glucose and insulin levels and hydrogen excretion in rats and healthy humans. British Journal of Nutrition, 118(2), 81–91. https://doi.org/https://doi.org/10.1017/S0007114517001866
- Oku, T. (1996). Oligosaccharides with beneficial health effects: A Japanese perspective. Nutrition Reviews, 54(11), S59–S66. https://doi.org/https://doi.org/10.1111/j.1753-4887.1996.tb03820.x
- Oku, T., Nakamura, M., Hashiguchi-Ishiguro, M., Tanabe, K., & Nakamura, S. (2009). Bioavailability and laxative threshold of 1-kestose in human adults. Dynamic Biochemistry, Process Biotechnologhy and Molecular Biology, 3, 90–95. http://www.globalsciencebooks.info/Online/GSBOnline/images/0906/DBPBMB_3(SI1)/DBPBMB_3(SI1)90-95o.pdf
- Oku, T., & Nakamura, S. (2002). Digestion, absorption, fermentation, and metabolism of functional sugar substitutes and their available energy. Pure and Applied Chemistry, 74(7), 1253–1261. https://doi.org/https://doi.org/10.1351/pac200274071253
- Oku, T., & Nakamura, S. (2014). Evaluation of the relative available energy of several dietary fiber preparations using breath hydrogen evolution in healthy humans. Journal of Nutritional Science and Vitaminology, 60(4), 246–254. https://doi.org/https://doi.org/10.3177/jnsv.60.246
- Oku, T., Nakamura, S., & Ichinose, M. (2005). Maximum permissive dosage of lactose and lactitol for transitory diarrhea, and utilizable capacity for lactose in japanese female adults. Journal of Nutritional Science and Vitaminology, 51(2), 51–57. https://doi.org/https://doi.org/10.3177/jnsv.51.51
- Roberfroid, M. B. (1999). Caloric value of inulin and oligofructose. The Journal of Nutrition, 129(7), 1436S–1437S. https://doi.org/https://doi.org/10.1093/jn/129.7.1436S
- Rumessen, J. J. (1992). Hydrogen and methane breath tests for evaluation of resistant carbohydrates. European Journal of Clinical Nutrition, 46(Suppl 2), S77–90.
- Storey, D. M., Koutsou, G. A., Lee, A., Zumbe, A., Olivier, P., Le Bot, Y., & Flourie, B. (1998). Tolerance and breath hydrogen excretion following ingestion of maltitol incorporated at two levels into milk chocolate consumed by healthy young adults with and without fasting. The Journal of Nutrition, 128(3), 587–592. https://doi.org/https://doi.org/10.1093/jn/128.3.587
- Suehiro, D., Okada, M., Fukami, K., Nakagawa, T., & Hayakawa, T. (2019). Maltobionic acid enhances intestinal absorption of calcium and magnesium in rats. Bioscience, Biotechnology, and Biochemistry, 83(9), 1766–1773. https://doi.org/https://doi.org/10.1080/09168451.2019.1611411
- Tachibe, M., Ohga, H., Nishibata, T., & Ebihara, K. (2011). Digestibility, fermentability, and energy value of highly cross-linked phosphate tapioca starch in men. Journal of Food Science, 76(6), H152–155. https://doi.org/https://doi.org/10.1111/j.1750-3841.2011.02247.x
- Tagami, H., Kishimoto, Y., Miyazato, S., Kitagawa, M., Hayashi, N., Yoshikawa, Y., Oga, H., Yamada, T., & Nishibata, T. (2012). Energy value evaluation of hydrogenated resistant maltodextrin. Bioscience, Biotechnology, and Biochemistry, 76(10), 1828–1834. https://doi.org/https://doi.org/10.1271/bbb.120106
- Tamura, A., Shiomi, T., Shigematsu, N., Tomita, F., & Hara, H. (2003). Evidence suggesting that difructose anhydride III is an indigestible and low fermentable sugar during the early stages after ingestion in humans. Journal of Nutritional Science and Vitaminology, 49(6), 422–427. https://doi.org/https://doi.org/10.3177/jnsv.49.422
- Tamura, A., Shiomi, T., Tamaki, N., Shigematsu, N., Tomita, F., & Hara, H. (2004). Comparative effect of repeated ingestion of difructose anhydride III and palatinose on the induction of gastrointestinal symptoms in humans. Bioscience, Biotechnology, and Biochemistry, 68(9), 1882–1887. https://doi.org/https://doi.org/10.1271/bbb.68.1882
- Tanabe, K., Ogawa, M., Nakamura, S., & Oku, T. (2018). Effects of ingestion of high-β-glucan barley bran cookies on postprandial glucose elevation and insulin secretion in healthy subject. Japanese Association for Dietary Fiber Research, 22(1), 21–28.
- Tanabe, K., Okuda, A., Nakamura, S., & Oku, T. (2020). Metabolic fate of newly developed nondigestible oligosaccharide, maltobionic acid in rats and humans. Food Science & Nutrition, 8(7), 3610–3616. https://doi.org/https://doi.org/10.1002/fsn3.1643