ABSTRACT
In this study, we evaluated the effects of immersion freezing (IF) with coolant and air freezing (AF) techniques with different storage temperature (−18°C and −30°C) on the quality of groupers following frozen storage. Water loss rate, total volatile basic nitrogen, and the K-value, were positively correlated with storage time. The pH first decreased and then increased. Destruction of the muscle fiber structure led to changes in grouper texture characteristics including hardness, cohesiveness, springiness, and chewiness. IF-30°C samples retained the highest quality after frozen storage, followed by IF-18°C samples and AF-18°C samples. Immersion freezing limited protein denaturation, decreased water loss, and contributed to the formation of uniformly distributed ice crystals, decreasing microstructure damage. Immersion freezing is a promising technique for preserving the quality of groupers, and the immersion coolant (propylene glycol, oligosaccharides and salts) used in this study has potential applications in the grouper processing industry.
RESUMEN
El presente estudio se propuso evaluar los efectos que las técnicas de congelación por inmersión (IF) con refrigerante y congelación por aire (AF) empleando diferentes temperaturas de almacenamiento (-18 °C y -30 °C) tienen en la calidad de los meros tras su almacenamiento congelado. Se constató que la tasa de pérdida de agua, el nitrógeno básico volátil total y el valor K se correlacionaron positivamente con el tiempo de almacenamiento. Asimismo, se comprobó que el pH primero disminuyó, para aumentar después. Además, la destrucción de la estructura de las fibras musculares provocó cambios en las características de textura del mero, como la dureza, la cohesión, la elasticidad y la masticabilidad. De esta forma, tras el almacenamiento en congelación, las muestras de IF-30 °C fueron las que conservaron la mayor calidad, seguidas por las muestras de IF-18 °C y las de AF-18 °C. Se comprobó que la congelación por inmersión limitó la desnaturalización de las proteínas, disminuyó la pérdida de agua y contribuyó a la formación de cristales de hielo uniformemente distribuidos, reduciendo los daños en la microestructura. Se concluye que la congelación por inmersión es una técnica prometedora para preservar la calidad de los meros, y el refrigerante empleado en la inmersión (propilenglicol, oligosacáridos y sales) realizada en este estudio posee aplicaciones potenciales en la industria de procesamiento del mero.
1. Introduction
Grouper (♀Epinephelus fuscoguttatus ×♂ Epinephelus lanceolatus) is a valuable fish that inhabits the bottom of warm offshore waters and is widely distributed across the Pacific and Indian Oceans (Chiu et al., Citation2012). The grouper is popular for its nutritional value and distinct flavor, as it is rich in various amino acids and polyunsaturated fatty acids required by the human body. However, because of its relatively low amount of connective tissue, tissue fragility, and high water content, it is vulnerable to microbial contamination during production and market circulation (X. Li et al., Citation2011). If not properly preserved, groupers become contaminated and deteriorate, resulting in a waste of resources and an environmental burden. Although grouper production has incresed year annually, but existing preservation technology does not meet the needs of the rapidly developing grouper industries. Previous studies demonstrated that freezing the water present in fish tissue increases the concentration of solutes (proteins, carbohydrates, fats, vitamins, and minerals), helping to destory the complex fish tissue homeostasis system (Yanco, Citation1981). Changes in the muscle fiber microenvironment alter cell membrane characteristics, thus affecting meat quality. Further, the size, position, and distribution of ice crystals in the frozen meat tissue are key determinants of the freezing rate (Wu et al., Citation2017). Therefore, a method for overcoming the technical bottleneck of traditional methods through developing low-cost and high-efficiency preservation technologies to extend the preservation period of products is important for the industry and research field.
Edible liquid soaking and freezing technology is an efficient technique that could shorten freezing time and have a positive influence on food quality. This approach uses direct heat exchange between the freezing liquid and refrigerant to achieve rapid freezing of food (Lucas & Raoult-Wack, Citation1998). To date, common freezing liquids include alcohols [ethanol (C2H6O) and propylene glycol (C3H8O2)], salts [(sodium chloride (NaCl) and calcium chloride (CaCl2)], and sugar solutions (Tsironi et al., Citation2009). Studies have shown that using edible liquid soaking and freezing can effectively preserve the integrity of the fish tissue structure (Liu et al., Citation2020). Because of the fluidity of the liquid, the heat transfer coefficient is high, demonstrating the potential of this method for food storage and preservation (Tello Alonso et al., Citation2011). In recent years, edible liquid soaking and freezing technology has been applied in food storage and preservation, including that of puffer fish (Yang et al., Citation2020), channel catfish (Xu et al., Citation2019), and white shrimp (Lopkulkiaert et al., Citation2009). However, its application in grouper preservation remains unclear. Qian et al. (Citation2018) observed that the liquid quick-freezing method could effectively ensure the integrity of the tissue structure, reduce the loss of juice after thawing, and maintain the quality of the frozen product. However, as 95% ethanol is applied, vacuum packaging is used to avoid the problem of high ethanol content in the food. The freezing liquid used in this study comprised edible ingredients with a considerably lower ethanol content (20% ethanol), allowing for favorable liquid fluidity and direct contact with the food.
To overcome the technical bottleneck of traditional freezing and maintain the freshness of grouper products, we evaluated immersion freezing (IF) coupled with coolant, an approach in the early stage of development for rapid freezing of groupers.
2. Materials and Methods
2.1. Materials
The grouper (♀Epinephelus fuscoguttatus ×♂ Epinephelus lanceolatus) (weight: 600–800 g) samples used in this study were supplied by a local grouper farm in Guangdong Province, China. Groupers were selected for uniformity of shape and pre-cooled to a temperature of 4°C. Thereafter, the groupers were packaged in polyethylene vacuum bags. The packaged groupers were randomly divided into three groups, with each group containing three groupers and subjected to three freezing treatments.
During preliminary research, our team selected food-grade refrigerants to study the lowest freezing point range of each principal component through single-factor experiments and then optimized the best refrigerants using the Box-Behnken response surface method to obtain a quick-freezing liquid formula with a low freezing point suitable for rapid freezing of aquatic products. The composition ratio of the quick-freezing liquid was 19.9% ethanol, 9.5% oligofructose, 3% citric acid, 5% calcium chloride, and 10% propylene glycol.
2.2. Freezing process
Immersion freezing (IF)-18°C (liquid freezing + −18°C storage): Groupers were placed in quick-freezing liquid (composed of food-grade propylene glycol, oligosaccharides, and salts) inside the WJ400H liquid-freezing machine (Wanjia Co., Ltd., Beijing, China). The temperature of the quick-freezing liquid in the machine was set to −40°C. The samples were removed after 55 min; when the core temperature of the fish reached −18°C, the samples were stored in a refrigerator at −18 ± 1°C.
IF-30°C (liquid freezing + −30°C): Groupers were placed in a quick-freezing liquid freezing machine pre-cooled to −40 ± 1°C and processed for 55 min. After the core temperature reached −18°C, the fish samples were removed and stored in a refrigerator at −30 ± 1°C.
Air freezing (AF)-18°C (air freezing + −18°C): Groupers were placed in a freezer at −20°C for 2 hours until the core temperature of the fish body was −18°C. The samples were then stored in a refrigerator at −18 ± 1°C.
Fish samples from the three groups were frozen and stored for 6 months. During the 6 months storage, the storage temperatures were controlled and monitored. Samples were removed at 30 days intervals and thawed at 4 ± 0.5°C for 12 hours for further analysis. The commonly employed air freezing method was used as a control treatment to compare quality changes during storage.
2.3. Thawing rate and thawing loss
The frozen samples were placed in a refrigerator at 4°C; the temperature was recorded every 20 s, and recording was suspended at 2°C. L (cm) represents the shortest distance between the surface and the center, and t (h) represents the thawing time of sample from 0 to 2°C. The thawing rate was calculated as follows:
Thawing rate (cm/h) = L (cm)/t (h)
Thawing loss was measured as described by Sun et al. (Sun et al., Citation2019). The specimens were weighed immediately before thawing W1(kg). Frozen samples were then thawed to a geometric center temperature of 4°C, dried with a paper towel, and weighed W2 (kg) immediately. Thawing loss was calculated as the percentage of weight loss before and after thawing according to the equation:
Thawing loss = (W1 – W2)/W1
2.4. pH determination
The pH was determined as described by Cai et al. (L. Y. Cai et al., Citation2020). Briefly, 10 g of minced fish was homogenized with 90 mL cold distilled water and incubated at room temperature (25°C) for 30 min. The pH of the supernatant was then measured using a digital pH meter (Mettler-Toledo GmbH, Columbus, OH, USA).
2.5. Total volatile basic nitrogen (TVB-N)
TVB-N was determined as described by F. Yang et al. (Citation2020) with a minor modification. Sample (5 g) was mixed with perchloric acid, and the supernatant was collected. The TVB-N content was then measured with a Kjeltec 2300 Atrauto Analyzer (Foss Corp., Copenhagen, Denmark). The supernatant was distilled and titrated automatically using the automatic nitrogen analyzer after adding 5 mL of 10 g/L MgO. TVB-N values are expressed in mg/100 g.
2.6. K-value
The extraction was performed in accordance with the method described by D. P. Li et al. (Citation2016) and the analysis was performed as described by Hao et al. (Hao et al., Citation2020). Briefly, 5 g homogenized samples were added to 20 mL of 10% perchloric acid solution, followed by centrifugation at 8 000 rpm for 10 min at 4°C. The supernatant was collected, and 10 mL of 5% perchloric acid solution was used to extract the test substance from the precipitate, followed by centrifugation at 8 000 rpm for 10 min at 4°C. The procedure was repeated once, and the supernatants were mixed. Next, 10 mol/L sodium hydroxide solution was used to adjust the pH of the extract to 6.0, followed by 1 mol/L sodium hydroxide solution to further adjust the pH to 6.0–6.4. The pH-adjusted solution was transferred to a pre-cooled volumetric flask and diluted to 50 mL with 4°C water. The solution was centrifuged at 8,000 rpm at 4°C and filtered through a 0.22-μm microporous membrane. The filtrate was analyzed using SHIMADZU-20A HPLC (Kyoto, Japan) with a C18 column (250 × 4.6 mm) (J. L. J. L. Liu et al., Citation2020). The filtrate (20 μL) was injected at a flow rate of 1.0 mL/min, and peaks were detected at 254 nm. The K-value was calculated as follows:
2.7. Ca2+-ATPase activity
Ca2+-ATPase activities were determined as described by Yasemi (Citation2017). A mixture containing 2.5 mL of mM Tris-HCl, 1.0 mL of 50 mM CaCl2, 1.0 mL of 4 M KCl, 1.5 mL of 6.67 mM ATP-Na2, and 4.0 mL of muscle fiber protease solution was placed in a water bath at 28°C for 30 min. The reaction was terminated by adding 1.0 mL of 15% trichloroacetic acid, followed by filtration through a membrane. The filtrate was collected, and its phosphorus content was determined using a Ca2+-ATPase kit.
2.8. Texture analysis
Texture profile analysis (TPA) was conducted to evaluate the sample texture characteristics, as described by Yu et al. (Yu et al., Citation2018) with minor modifications. The samples were cut into small pieces (20 × 20 × 10 mm) and analyzed using a CT 3–4500 texture analyzer (Brookfield Engineering, Middleborough, MA, USA) with a cylindrical probe P/5. The compression strain was set at 50%, and the trigger force was 5 g. The pretest, test, and post-test speed were 30 mm/min. Hardness, cohesiveness, springiness, and chewiness were assessed in three biological replicates.
2.9. Microstructure of grouper samples
Grouper microstructure was analyzed as described by Choi et al. (Citation2017). with minor modifications. The groupers were filleted (10 × 10 × 3 mm), fixed using 10% methanol for 48 h, and trimmed to a size of 3 × 3 × 3 mm. All samples were then dehydrated by gradient ethanol treatment (30%, 50%, 70%, 80%, 90%, and 100%), cleared in xylene for 4 h, immersed in a paraffin bath for 2 h, embedded in paraffin, and cut into slices (10 μm). The slices were mounted on glass plates and incubated in an oven at 70°C for 1–2 h. Elution using xylene was performed twice for 40 min, followed by elution using ethanol also performed twice. Finally, the slices were stained with eosin solution for 10–20 s, decolorized with 95% ethanol for a few seconds, dehydrated with absolute ethanol for 2–3 min, and then treated with xylene for 40 min. All prepared slices were observed under a microscope (Olympus, Tokyo, Japan).
2.10. Statistical analysis
All results were presented as the averages of three biological replicates. One-way analysis of variance was performed using SPSS version 19.0 software (SPSS, Inc., Chicago, IL, USA). MetaboAnalyst 4.0 was employed for principal component analysis (PCA). Heat maps were plotted with the R software (v3.1.1, R Foundation for Statistical Computing, Vienna, Austria).
3. Results and Discussion
3.1. Thawing rate and water loss rate
The thawing rate is closely related to the thawing time, which affects the texture of the fish. As shown in , the thawing rates of groupers frozen via different freezing methods decreased with prolonged frozen storage. This may result from changes in the microstructure of frozen grouper as the storage time increased. The curves indicated that the thawing rate of the samples in the AF-18°C group was significantly higher than the IF-30°C and IF-18°C samples, with a lower IF temperature leading to a lower thawing rate. The final temperature of frozen samples was associated with the thawing rate owing to the temperature range. As the frozen storage time increased, the thawing rate of samples from all three groups gradually decreased. The thawing rate of IF-30°C, IF-18°C, and AF-18°C decreased by 42.18%, 38.10%, and 32.34%, respectively, compared with the initial thawing rate. The thawing rate decreased rapidly initially and then decreased further at a lower rate, indicating that the groupers were deeply frozen during extended storage.
Figure 1. Thawing rate (a) and water loss rate (b) of grouper fillets after different treatment during storage. IF-18 °C, fillets were subjected to immersion freezing and stored at -18 °C; IF-30 °C, fillets were subjected to immersion freezing and stored at -30 °C; AF-18 °C, fillets were subjected to air freezing and stored at -18 °C. Different letters indicate significant differences (P < 0.05).
Figura 1. Tasa de descongelación (a) y tasa de pérdida de agua (b) de los filetes de mero tras la aplicación de diferentes tratamientos durante el almacenamiento. IF-18 °C, los filetes fueron sometidos a congelación por inmersión y almacenados a -18 °C; IF-30 °C, los filetes fueron sometidos a congelación por inmersión y almacenados a -30 °C; AF-18 °C, los filetes fueron sometidos a congelación por aire y almacenados a -18 °C. Las letras distintas indican diferencias significativas (P < 0.05)
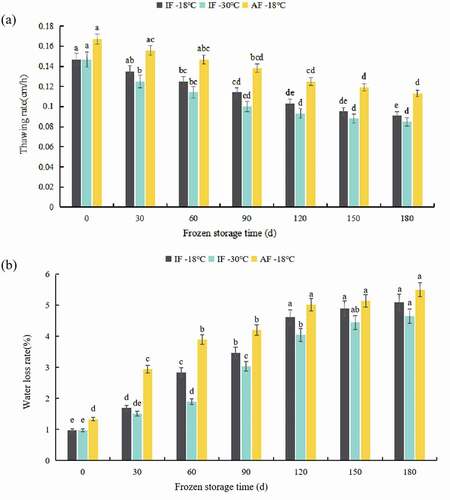
Thawing loss is caused by water and water soluble proteins leakage that affects the appearance of products and leads to the loss of nutrients from fish, thus reducing its commercial value (Sun et al., Citation2019). The loss of water is closely related to the thawing rate, ice crystal formation, and structural integrity of muscle fibers (M. C. Zhang et al., Citation2019). As shown in , the thawing loss of groupers in all groups gradually increased with extended storage time. Increased thawing loss has been considered an effect of structural changes, including myosin denaturation, shrinkage of the myofilament lattice, and increased extracellular space (Luan et al., Citation2018). The thawing loss of the AF-18°C groupers was 37.11% higher than IF samples (IF-18°C and IF-30°C) at 0 days. The high thawing loss observed in the AF-18°C group during frozen storage may be attributed to the low freezing rate leading to the formation of large ice crystals, which destroy the tight muscle tissue structure of fish (Dongni Li et al., Citation2020). Compared with air freezing, immersion freezing exhibited a faster freezing speed, resulting in small and uniform ice crystals that caused minor damage to the cell structure. Further, the thawing loss of AF-18°C samples increased at a higher rate than for the IF-30°C and IF-18°C samples from the beginning of the storage up to 60 days. Thereafter, the thawing loss of all groups remained constant from 120 to 180 days. At the end of the storage, AF-18°C samples exhibited the highest thawing loss, followed by IF-18°C and IF-30°C samples. These results suggest that IF-30°C groupers had the highest water-holding capacity, confirming the results of the study by Goncalves and Ribeiro (Citation2008), which indicated that faster freezing resulted in fewer losses during thawing. In contrast, slow freezing rates help form larger ice crystals, which cause greater structural cryodamage to muscle cells and protein denaturation (C. L. Kong et al., Citation2016). Different freezing treatments and freezing temperatures significantly influenced thawing loss in groupers. Shi et al. (Citation2018) reported that perches exhibited high loss of moisture with increasing storage temperatures from −80°C to −18°C.
3.2. pH
The pH values can be influenced by anaerobic glycolysis and autolytic and microbial reactions during the freezing process and storage, thus, it is considered as a parameter for evaluating fish spoilage (D. Liu et al., Citation2013). The effects of different treatments on the pH of groupers during frozen storage are shown in . On day 0, there was no significant difference in the pH among groups, as the pH value of grouper samples from all the three groups was 6.9. Thereafter, the pH of groupers from all groups initially decreased, followed by an increase. In the first 30 days of storage, AF-18°C samples exhibited a significant decline of 3.04%, from pH 6.9 to 6.69. This initial reduction in pH was attributed to the formation of lactic acid, caused primarily by postmortem glycogenolysis in fish (Bahmani et al., Citation2011). In addition, the fermentation of carbohydrates caused by bacteria contributes to the accumulation of organic acids in fish (L. Cai et al., Citation2014). After 30 days of storage, an increased pH resulted from the production of spoilage-related alkaline metabolites (Hong et al., Citation2013), such as trimethylamine and ammonia, produced by protein degradation and microbial activity (Subramanian, Citation2007). From 30 to 180 days, the increased rates of pH in IF-30°C, IF-18°C, and AF-18°C groupers were 9.06%, 9.52%, and 10.76%, respectively, indicating that low temperatures limited protein denaturation and fish spoilage. With the extension of the storage, the increased rates of pH slowed down. The pH of AF-18°C groupers was the highest at 180 days; this may be related to the destruction of the cell structure and leakage of intracellular constituents because of the formation of large ice crystals by air freezing.
Figure 2. pH of grouper fillets after different freezing treatments during storage. Statistical analysis was performed via one-way analysis of variance with Duncan’s test. Different letters indicate significant differences (P < 0.05) during storage. IF-18 °C, fillets were subjected to immersion freezing and stored at -18 °C; IF-30 °C, fillets were subjected to immersion freezing and stored at -30°C; AF-18°C, fillets were subjected to air freezing and stored at -18 °C. Different letters indicate significant differences (P < 0.05).
Figura 2. pH de los filetes de mero después de diferentes tratamientos de congelación durante el almacenamiento. El análisis estadístico se realizó mediante un análisis de varianza de una vía con la prueba de Duncan. Las letras distintas indican diferencias significativas (P < 0.05) durante el almacenamiento. IF-18 °C, los filetes fueron sometidos a congelación por inmersión y almacenados a -18 °C; IF-30 °C, los filetes fueron sometidos a congelación por inmersión y almacenados a -30°C; AF-18°C, los filetes fueron sometidos a congelación por aire y almacenados a -18 °C. Las letras distintas indican diferencias significativas (P < 0.05)
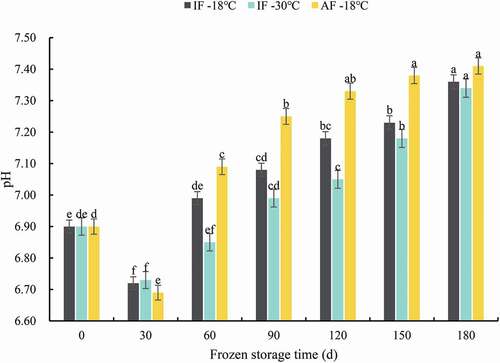
3.3. TVB-N
Total volatile basic nitrogen (TVB-N) is generated by protein and nitrogenous non-protein degradation caused enzymatic and microbial activity that is used for assessing spoilage of fish products (Gao et al., Citation2014). The TVB-N content of groupers from the three different treatment groups is shown in . There was no significant difference in the initial TVB-N between groups, indicating that freezing treatments had no noticeable effect on the initial TVB-N of the groupers and reflected grouper freshness. This observation was attributed to the low temperature-mediated inhibition of microbial growth. The TVB-N values of samples from the three treatment groups gradually increased with storage time, and the TVB-N values of AF-18°C samples were higher than others during storage. The TVB-N values for IF-30°C, IF-18°C, and AF-18°C were 18.603, 20.020, and 22.635 mg/100 g at 180 days, respectively. During the freezing process, the low temperature of the quick-freezing liquid led to the inactivation of microorganisms intolerant to low temperatures, reducing the microorganism count and delaying the spoilage and deterioration of groupers during the storage. TVB-N can be increased by microbial and autolytic activities and the complete microbial reduction of trimethylamine oxide to trimethylamine (Hong et al., Citation2013). Low temperature can effectively inhibit the activity of spoilage bacteria and autolytic enzymes, slowing the spoilage process and production of volatile compounds (Soares et al., Citation2015). In this study, the TVB-N content was well within acceptable limits. These results indicate that cryogenic freezing effectively maintained sample quality by inhibiting endogenous enzymes and microbial activity.
Figure 3. TVB-N (a) and K-value (b) of grouper fillets after freezing treatments during storage. Statistical analysis was performed via one-way analysis of variance with Duncan’s test. Different letters indicate significant differences (P < 0.05) during storage. IF-18 °C, fillets were subjected to immersion freezing and stored at -18 °C; IF-30 °C, fillets were subjected to immersion freezing and stored at -30 °C; AF-18 °C, fillets were subjected to air freezing and stored at -18 °C. Different letters indicate significant differences (P < 0.05).
Figura 3. TVB-N (a) y valor K (b) de los filetes de mero tras los tratamientos de congelación durante el almacenamiento. El análisis estadístico se realizó mediante un análisis de varianza de una vía con la prueba de Duncan. Las letras distintas indican diferencias significativas (P < 0.05) durante el almacenamiento. IF-18 °C, los filetes fueron sometidos a congelación por inmersión y almacenados a -18 °C; IF-30 °C, los filetes fueron sometidos a congelación por inmersión y almacenados a -30 °C; AF-18 °C, los filetes fueron sometidos a congelación por aire y almacenados a -18 °C. Las letras distintas indican diferencias significativas (P < 0.05)
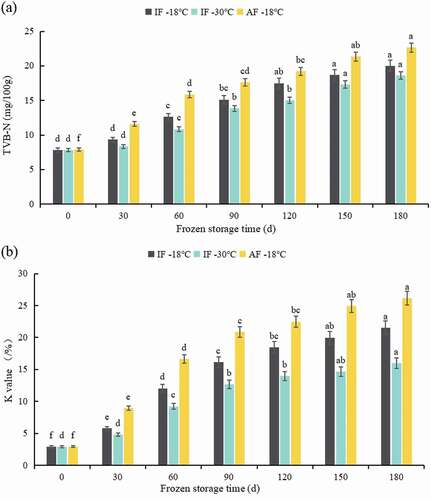
3.4. K-value
The K-value, as an essential indicator for evaluating the freshness of fish, is related to the postmortem ATP breakdown in fish. (Hong et al., Citation2017). This value is the ratio of the sum of inosine and hypoxanthine to the sum of all the ATP degradation products (Lakshmisha et al., Citation2008). As shown in , the K-value of groupers from the three freezing treatment groups increased progressively with storage time. During frozen storage, the K-values in the IF-30°C and IF-18°C groups were lower than that in the AF-18°C group. This is because of the high freezing rate of liquid freezing, which reduces the activity of 5-nucleotidase, inhibiting the breakdown of inosine monophosphate (L. L. Cai et al., Citation2014). The degradation of inosine monophosphate into inosine and hypoxanthine leads to loss of the umami components of fish and decreased freshness (Hong et al., Citation2017). The K-values of the IF-30°C and IF-18°C samples showed no significant difference before 30 days; this may be because of the increase in the K-value during the early storage period being caused by enzymatic degradation (Lee & Park, Citation2016). At 90 days, the K-values of IF-30°C, IF-18°C, and AF-18°C increased from 2.94% to 12.69%, 16.17%, and 20.87%, respectively. Based on the evaluation criteria (Yu et al., Citation2018), IF-30°C and IF-18°C samples were fresh, whereas AF-18°C samples were moderately fresh at 90 days. These results suggest that IF and cryogenic storage limit ATP degradation and preserve the freshness and quality of fish.
3.5. Ca2+-ATPase activity
Ca2+-ATPase activity has been widely used as an indicator of fish myofibrillar protein denaturation during frozen storage, because it is associated with the proteolysis of the myosin molecule (Zhao et al., Citation2013). As shown in , the Ca2+-ATPase activity of samples from all groups decreased continuously with the storage duration. Decreases in Ca2+-ATPase activity are caused by various microstructural changes in myofibrillar protein (B. B. Zhang et al., Citation2015). A sharp decrease in Ca2+-ATPase activity was observed during the first 30 days in all three treatment groups. Similar results were reported by Qian et al. (Citation2018), who suggested that the decrease in Ca2+-ATPase activity in the initial stage occurs mainly because of the oxidation of sulfhydryl groups and denaturation of myofibrils caused by temperature changes. At 30 days, Ca2+-ATPase activity in IF-18°C, IF-30°C, and AF-18°C samples decreased to 32.55%, 30.23%, and 44.19%, respectively. The relatively high Ca2+-ATPase activity in IF-18°C and IF-30°C groupers can be explained by the myofibrillar protein structure being well protected by immersion freezing. Immersion freezing with a high freezing rate reduced damage to the microstructure because of the formation of smaller and more evenly distributed ice crystals. By the end of frozen storage, Ca2+-ATPase activity decreased from 0.43 to 0.135 U/mg protein in IF-18°C, 0.160 U/mg protein in IF-30°C, and 0.093 U/mg protein in AF-18°C groupers. IF-30°C groupers exhibited the highest Ca2+-ATPase activity. The sulfhydryl group of cysteine is central to Ca2+-ATPase activity, and low temperatures can inhibit the oxidation of sulfhydryl groups (C. L. Kong et al., Citation2016). These results indicate that IF-30°C treatment was better for maintaining Ca2+-ATPase activity.
Figure 4. Ca2+-ATP activity of grouper fillets after freezing treatment during storage. Statistical analysis was performed via one-way analysis of variance with Duncan’s test. Different letters indicate significant differences (P < 0.05) during storage. IF-18 °C, fillets were subjected to immersion freezing and stored at -18 °C; IF-30 °C, fillets were subjected to immersion freezing and stored at -30 °C; AF-18 °C, fillets were subjected to air freezing and stored at -18 °C. Different letters indicate significant differences (P < 0.05).
Figura 4. Actividad Ca2+-ATP de los filetes de mero tras el tratamiento de congelación durante el almacenamiento. El análisis estadístico se realizó mediante un análisis de varianza de una vía con la prueba de Duncan. Las letras distintas indican diferencias significativas (P < 0.05) durante el almacenamiento. IF-18 °C, los filetes fueron sometidos a congelación por inmersión y almacenados a -18 °C; IF-30 °C, los filetes fueron sometidos a congelación por inmersión y almacenados a -30 °C; AF-18 °C, los filetes fueron sometidos a congelación por aire y almacenados a -18 °C. Las letras distintas indican diferencias significativas (P < 0.05)
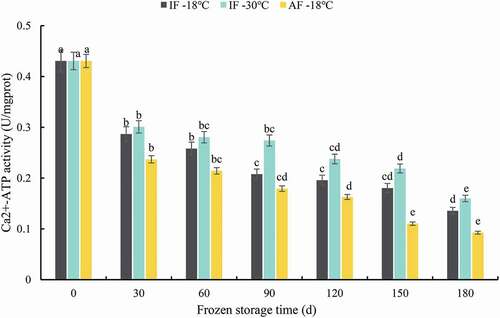
3.6. Microstructure of muscle fibers
Frozen aquatic product quality is directly influenced by the size and distribution of ice crystals, which are essential factors affecting microstructural integrity (Louly et al., Citation2011). presents cross-sections and longitudinal sections of the frozen muscle tissue from different freezing treatment groups. The cross-section of fresh groupers exhibited regular intact muscle fibers and smooth endomysium, in accordance with observations in obscure pufferfish (F. Yang et al., Citation2020) and rainbow trout (Hafezparast-Moadab et al., Citation2018). As observed in the longitudinal sections of fresh samples, the perimysium of fresh groupers was neatly arranged and appeared compact and dense. During the initial stage of storage, the microstructure of groupers subjected to immersion freezing did not significantly differ from that of fresh samples, whereas the tissue structure of samples from the AF-18°C group was looser, and the gaps between the muscle fibers were greater than those of the samples from the other treatment groups. The better cellular structure observed in immersion freezing samples compared with their air freezing counterparts suggested that immersion freezing could effectively alleviate mechanical damage to the texture of groupers. Similar results were reported by Xu et al. (Citation2019). After up to 180 days of storage, the microstructure was damaged, and cellular integrity was seriously compromised in all three groups, suggesting that the freezing time was related to the size and distribution of ice crystals. A shorter freezing time resulted in smaller ice crystals. AF-18°C grouper muscle fibers were damaged and deformed with visible detachments and cracks between the muscle fibers. In IF samples, relatively small ice crystals were observed. Immersion freezing is a faster freezing treatment compared to air freezing, suggesting that rapid freezing and low temperature can reduce texture damage because of more intensive nucleation and the formation of numerous small ice crystals. The microstructure results were consistent with the changes in the thawing rate, thawing loss, and texture characteristics of the groupers. Immersion freezing is a promising technology for controlling the formation of ice crystals during the freezing and preservation of grouper tissue (Dongmei Li et al., Citation2018).
Figure 5. Microscope images of grouper fillets after freezing treatments during storage. IF-18 °C, fillets were subjected to immersion freezing and stored at -18 °C; IF-30 °C, fillets were subjected to immersion freezing and stored at -30 °C; AF-18 °C, fillets were subjected to air freezing and stored at -18 °C.
Figura 5. Imágenes de microscopio de los filetes de mero después de los tratamientos de congelación durante el almacenamiento. IF-18 °C, los filetes fueron sometidos a congelación por inmersión y almacenados a -18 °C; IF-30 °C, los filetes fueron sometidos a congelación por inmersión y almacenados a -30 °C; AF-18 °C, los filetes fueron sometidos a congelación por aire y almacenados a -18 °C
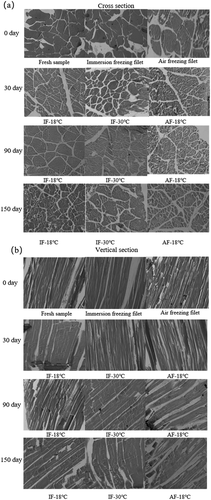
3.7. Texture characteristics
Texture characteristics of samples with different treatment were analyzed by texture profile analysis (TPA), including hardness, cohesiveness, springiness and chewiness. Texture, which is mainly influenced by the freezing rate, is directly associated with the product acceptability by consumers (Liang et al., Citation2015).Moreover, the texture of frozen fish is influenced by the breakdown of muscle fiber and protein denaturation (Hafezparast-Moadab et al., Citation2018). Changes in the texture characteristics of grouper samples during frozen storage are shown in . Changes followed the same trend as hardness, cohesiveness, springiness, and chewiness values decreased with longer storage time. Hardness decreased in all three groups after 30 days of frozen storage, particularly in AF-18°C samples, and continued to decrease steadily. At the end of frozen storage, the hardness values of IF-30°C, IF-18°C, and AF-18°C groupers were 52.41, 49.1, and 38.3 g, accounting for 54.08%, 50.67%, and 39.24% of the hardness of the fresh samples, respectively. Protein degradation resulting in a decreased intermuscular cell-binding force and loose spatial structure of proteins may contribute to decreased hardness (Wei et al., Citation2021). IF-30°C groupers showed higher hardness values than IF-18°C and AF-18°C groupers, indicating that high freezing rates and low storage temperatures inhibit microbial and enzymatic activity to preserve hardness. Similar results were obtained by F. Yang et al. (Citation2020), who found that immersion freezing, particularly at −30°C, was more efficient for preserving the hardness of fish fillets. Moreover, the formation of large extracellular ice crystals and shrinkage of cells reportedly induce tissue deformation, resulting in reduced hardness (Alizadeh et al., Citation2007). Consistent with the results of the analysis of other texture characteristics, immersion freezing better maintained the integrity of the muscle structure.
Figure 6. Heat map visualization of different texture characteristics after different freezing treatment and storage conditions. IF-18 °C, fillets were subjected to immersion freezing and stored at -18 °C; IF-30 °C, fillets were subjected to immersion freezing and stored at -30 °C; AF-18 °C, fillets were subjected to air freezing and stored at -18 °C.
Figura 6. Visualización del mapa de calor de las diferentes características de textura después de distintos tratamientos de congelación y condiciones de almacenamiento. IF-18 °C, los filetes fueron sometidos a congelación por inmersión y almacenados a -18 °C; IF-30 °C, los filetes fueron sometidos a congelación por inmersión y almacenados a -30 °C; AF-18 °C, los filetes fueron sometidos a congelación por aire y almacenados a -18 °C
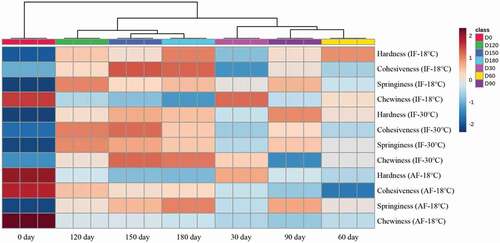
IF-30°C samples exhibited higher cohesiveness than the IF-18°C and AF-18°C samples, whereas the AF-18°C samples had the lowest cohesiveness. Cohesiveness decreased slowly after 30 days in all three groups, and the cohesiveness of IF groupers was similar but significantly higher than AF-18°C grouper. The results indicate that immersion freezing preserves the cohesiveness of groupers during frozen storage. The reduction in cohesiveness may be attributed to the decomposition of protein and fat caused by damage to the structural integrity of muscle fibers (Wang et al., Citation2020).
The springiness of IF-30°C, IF-18°C, and AF-18°C samples decreased from 0.7 to 0.45, 0.41, and 0.37, respectively. Correspondingly, the decrease rates of IF-30°C, IF-18°C, and AF-18°C were 35.71%, 41.43%, and 47.14%. Springiness was related to the gel strength of muscles. The reduction of gel strength leads to a decrease in springiness because of protein denaturation caused by the freezing process (Wang et al., Citation2020).
Chewiness is an important indicator of texture in fish. Protein degradation and fat oxidation during freezing and drip loss during thawing affect texture and chewiness. AF-18°C groupers exhibited the lowest chewiness and highest decrease rate. These results suggest that cryogenic storage and a high freezing rate were beneficial for preserving grouper chewiness. Thus, immersion freezing technology had a greater protective effect on the texture of groupers after freezing and long-term frozen storage.
3.8. Principal component analysis (PCA)
PCA uses linear transformation to convert variables to compress data and extract principal components (PCs). Thus, we employed PCA to gain insight into the correlation and differences of different physicochemical properties. PCA reduced the initial variables to five PCs, which explained 99.2% of the total variance. As shown in , PC1 (76.7%) and PC2 (16.2%) explained 92.9% of the total variance, indicating a highly significant correlation between physicochemical properties of groupers and frozen storage. A two-dimensional space with the first two PCs as loading factors was used to compare different physicochemical properties between treatments. The group comprised J-1, J-2, and J-3, suggesting that different storage temperatures have a significant impact on Ca2+-ATPase activity in groupers during storage. Hardness was negatively correlated with PC1, indicating that hardness decreased with longer storage times because of the reduction in the water-holding capacity and destruction of muscle tissues (Dongni Li et al., Citation2020). In addition, the change in hardness mainly occurred during the early storage period (0–30 days); this is in agreement with the results of texture analysis. The group included C-1, C-2, and C-3 with some distances, suggesting that the effect of freezing treatment on the pH of frozen groupers was evident, particularly during the 60-day storage period. The strongest correlation was observed between the water loss rate and TVB-N. During the freezing process, the structure of muscle tissue was compromised, resulting in water loss. Leakage of intracellular fluid provides a favorable environment for the growth of microorganisms (Duun & Rustad, Citation2007). Bacteria decompose protein to produce alkaline nitrogen-containing volatile substances, such as ammonia and histamine, resulting in an increase in the TVB-N content. shows that the increase in the water loss rate, TVB-N, and K-value was highest following 180-day storage. Additionally, based on the storage time, the PCA bi-plot revealed that properties mainly clustered in the second and fourth quadrant. In contrast, the properties of H-1, 2, 3 and F-1, 2, 3 were distributed in the third quadrant, indicating these two physicochemical properties differed from the other properties.
Figure 7. Principal component analysis (PCA) score plot of grouper fillets with different physicochemical properties (a). PCA bi-plot of grouper fillets with different physicochemical properties (b).
Figura 7. Gráfico de puntuación del análisis de componentes principales (PCA) de los filetes de mero con diferentes propiedades fisicoquímicas (a). Biplot del PCA de los filetes de mero con diferentes propiedades fisicoquímicas (b)
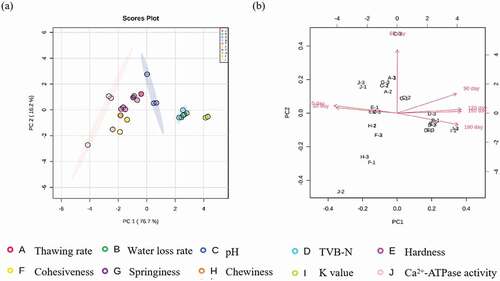
4. Conclusion
In this study, the effects of immersion freezing and air freezing on the quality of groupers were evaluated. The lower TVB-N and K-value following immersion treatment suggested that immersion freezing effectively inhibited the growth of microorganisms and delayed the contamination and deterioration of groupers. IF-30°C groupers exhibited minimal damage to the spatial structure of myosin and higher Ca2+-ATPase activity compared to the other freezing treatment groups. The texture characteristics (hardness, cohesiveness, springiness and chewiness) showed a decreased trends during frozen storage and IF-30°C groupers has the least changes compared with other two groups. PCA indicated that Ca2+-ATPase activity was influenced by storage temperature and water loss rate and TVB-N had the strongest certain correlation. In conclusion, immersion freezing is a promising technique for preserving the quality of groupers, and the immersion coolant used in this study has potential applications in the grouper processing industry.
Acknowledgments
This work was supported by the National Key R&D Program of China (2019YFD0901903), China Agriculture Research System of MOF and MARA (CARS-47), National Natural Science Foundation of China (32001733), Guangdong Basic and Applied Basic Research Foundation (2019A1515111158), Special Scientific Research Funds for Central Non-profit Institutes, Chinese Academy of Fishery Sciences (2020TD69), Fund of Key Laboratory of Aquatic Product Processing, Ministry of Agriculture and Rural Affairs, China (NYJG202007).
Disclosure statement
The authors declare that they do not have any conflicts of interest.
References
- Alizadeh, E., Chapleau, N., de Lamballerie, M., & Le-Bail, A. (2007). Effect of different freezing processes on the microstructure of Atlantic salmon (Salmo salar) fillets. Innovative Food Science & Emerging Technologies, 8(4), 493–499. https://doi.org/https://doi.org/10.1016/j.ifset.2006.12.003
- Bahmani, Z. A., Rezai, M., Hosseini, S. V., Regenstein, J. M., Bohme, K., Alishahi, A., & Yadollahi, F. (2011). Chilled storage of golden gray mullet (Liza aurata). Lwt-Food Science and Technology, 44(9), 1894–1900. https://doi.org/https://doi.org/10.1016/j.lwt.2011.01.009
- Cai, L., Wu, X., Li, X., Zhong, K., Li, Y., & Li, J. (2014). Effects of different freezing treatments on physicochemical responses and microbial characteristics of Japanese sea bass (Lateolabrax japonicas) fillets during refrigerated storage. LWT - Food Science and Technology, 59(1), 122–129. https://doi.org/https://doi.org/10.1016/j.lwt.2014.04.062
- Cai, L. Y., Wan, J. L., Li, X. X., & Li, J. R. (2020). Effects of different thawing methods on physicochemical properties and structure of largemouth bass (Micropterus salmoides) [Article]. Journal of Food Science, 85(3), 582–591. https://doi.org/https://doi.org/10.1111/1750-3841.15029
- Chiu, T.-H., Su, Y.-C., Pai, J.-Y., & Chang, H.-C. (2012). Molecular markers for detection and diagnosis of the giant grouper (Epinephelus lanceolatus). Food Control, 24(1), 29–37. https://doi.org/https://doi.org/10.1016/j.foodcont.2011.08.033
- Choi, E. J., Park, H. W., Chung, Y. B., Park, S. H., Kim, J. S., & Chun, H. H. (2017). Effect of tempering methods on quality changes of pork loin frozen by cryogenic immersion. Meat Science, 124(2), 69–76. https://doi.org/https://doi.org/10.1016/j.meatsci.2016.11.003
- Duun, A. S., & Rustad, T. (2007). Quality changes during superchilled storage of cod (Gadus morhua) fillets. Food Chemistry, 105(3), 1067–1075. https://doi.org/https://doi.org/10.1016/j.foodchem.2007.05.020
- Gao, M. S., Feng, L. F., Jiang, T. J., Zhu, J. L., Fu, L. L., Yuan, D. X., & Li, J. R. (2014). The use of rosemary extract in combination with nisin to extend the shelf life of pompano (Trachinotus ovatus) fillet during chilled storage. Food Control, 37(3), 1–8. https://doi.org/https://doi.org/10.1016/j.foodcont.2013.09.010
- Goncalves, A. A., & Ribeiro, J. L. D. (2008). Optimization of the freezing process of red shrimp (Pleoticus muelleri) previously treated with phosphates. International Journal of Refrigeration-Revue Internationale Du Froid, 31(7), 1134–1144. https://doi.org/https://doi.org/10.1016/j.ijrefrig.2008.03.005
- Hafezparast-Moadab, N., Hamdami, N., Dalvi-Isfahan, M., & Farahnaky, A. (2018). Effects of radiofrequency-assisted freezing on microstructure and quality of rainbow trout (Oncorhynchus mykiss) fillet. Innovative Food Science & Emerging Technologies, 47(6), 81–87. https://doi.org/https://doi.org/10.1016/j.ifset.2017.12.012
- Hao, R., Pan, J., Tilami, S. K., Shah, B. R., & Mraz, J. (2020). Post-mortem quality changes of common carp (Cyprinus carpio) during chilled storage from two culture systems. Journal of Science of Food and Agriculture, 101(1), 91–100. https://doi.org/https://doi.org/10.1002/jsfa.10618
- Hong, H., Luo, Y., Zhou, Z., Bao, Y., Lu, H., & Shen, H. (2013). Effects of different freezing treatments on the biogenic amine and quality changes of bighead carp (Aristichthys nobilis) heads during ice storage. Food Chemistry, 138(2), 1476–1482. https://doi.org/https://doi.org/10.1016/j.foodchem.2012.11.031
- Hong, H., Regenstein, J. M., & Luo, Y. K. (2017). The importance of ATP-related compounds for the freshness and flavor of post-mortem fish and shellfish muscle: A review. Critical Reviews in Food Science and Nutrition, 57(9), 1787–1798. https://doi.org/https://doi.org/10.1080/10408398.2014.1001489
- Kong, C. H. Z., Hamid, N., Liu, T. T., & Sarojini, V. (2016). Effect of Antifreeze Peptide Pretreatment on Ice Crystal Size, Drip Loss, Texture, and Volatile Compounds of Frozen Carrots. Journal of Agricultural and Food Chemistry, 64(21), 4327–4335. https://doi.org/https://doi.org/10.1021/acs.jafc.6b00046
- Kong, C. L., Wang, H. Y., Li, D. P., Zhang, Y. M., Pan, J. F., Zhu, B. W., & Luo, Y. K. (2016). Quality changes and predictive models of radial basis function neural networks for brined common carp (Cyprinus carpio) fillets during frozen storage. Food Chemistry, 201(12), 327–333. https://doi.org/https://doi.org/10.1016/j.foodchem.2016.01.088
- Lakshmisha, I. P., Ravishankar, C. N., Ninan, G., Mohan, C. O., & Gopal, T. K. S. (2008). Effect of Freezing Time on the Quality of Indian Mackerel (Rastrelliger kanagurta) during Frozen Storage. Journal of Food Science, 73(7), S345–S353. https://doi.org/https://doi.org/10.1111/j.1750-3841.2008.00876.x
- Lee, J., & Park, J. W. (2016). Pacific whiting frozen fillets as affected by postharvest processing and storage conditions. Food Chemistry, 201(12), 177–184. https://doi.org/https://doi.org/10.1016/j.foodchem.2016.01.083
- Li, D., Zhao, H., Muhammad, A. I., Song, L., Guo, M., & Liu, D. (2020). The comparison of ultrasound-assisted thawing, air thawing and water immersion thawing on the quality of slow/fast freezing bighead carp (Aristichthys nobilis) fillets. Food Chemistry, 320(19), 126614. https://doi.org/https://doi.org/10.1016/j.foodchem.2020.126614
- Li, D., Zhu, Z., & Sun, D.-W. (2018). Effects of freezing on cell structure of fresh cellular food materials: A review. Trends in Food Science & Technology, 75(5), 46–55. https://doi.org/https://doi.org/10.1016/j.tifs.2018.02.019
- Li, D. P., Qin, N., Zhang, L. T., Lv, J., Li, Q. Z., & Luo, Y. K. (2016). Effects of different concentrations of metal ions on degradation of adenosine triphosphate in common carp (Cyprinus carpio) fillets stored at 4 °C: An in vivo study. Food Chemistry, 211(32), 812–818. https://doi.org/https://doi.org/10.1016/j.foodchem.2016.05.120
- Li, X., Li, J., Zhu, J., Wang, Y., Fu, L., & Xuan, W. (2011). Postmortem changes in yellow grouper (Epinephelus awoara) fillets stored under vacuum packaging at 0°C. Food Chemistry, 126(3), 896–901. https://doi.org/https://doi.org/10.1016/j.foodchem.2010.11.071
- Liang, D. W., Lin, F. Y., Yang, G. M., Yue, X. J., Zhang, Q. K., Zhang, Z. Q., & Chen, H. B. (2015). Advantages of immersion freezing for quality preservation of litchi fruit during frozen storage. LWT - Food Science and Technology, 60(2), 948–956. https://doi.org/https://doi.org/10.1016/j.lwt.2014.10.034
- Liu, D., Liang, L., Xia, W., Regenstein, J. M., & Zhou, P. (2013). Biochemical and physical changes of grass carp (Ctenopharyngodon idella) fillets stored at −3 and 0°C. Food Chemistry, 140(1–2), 105–114. https://doi.org/https://doi.org/10.1016/j.foodchem.2013.02.034
- Liu, J. L., Lan, W. Q., Sun, X. H., & Xie, J. (2020). Effects of chitosan grafted phenolic acid coating on microbiological, physicochemical and protein changes of sea bass (Lateolabrax japonicus) during refrigerated storage. Journal of Food Science, 85(8), 2506–2515. https://doi.org/https://doi.org/10.1111/1750-3841.15329
- Liu, S. L., Zeng, X. H., Zhang, Z. Y., Long, G. Y., Lyu, F., Cai, Y. P., Liu, J. H., & Ding, Y. T. (2020). Effects of Immersion Freezing on Ice Crystal Formation and the Protein Properties of Snakehead (Channa argus). Foods, 9(4), 12. https://doi.org/https://doi.org/10.3390/foods9040411
- Lopkulkiaert, W., Prapatsornwattana, K., & Rungsardthong, V. (2009). Effects of sodium bicarbonate containing traces of citric acid in combination with sodium chloride on yield and some properties of white shrimp (Penaeus vannamei) frozen by shelf freezing, air-blast and cryogenic freezing. LWT - Food Science and Technology, 42(3), 768–776. https://doi.org/https://doi.org/10.1016/j.lwt.2008.09.019
- Louly, A., Gaydou, E. M., & El Kebir, M. V. O. (2011). Muscle lipids and fatty acid profiles of three edible fish from the Mauritanian coast: Epinephelus aeneus, Cephalopholis taeniops and Serranus scriba. Food Chemistry, 124(1), 24–28. https://doi.org/https://doi.org/10.1016/j.foodchem.2010.05.097
- Luan, L. L., Wang, L. P., Wu, T. T., Chen, S. G., Ding, T., & Hu, Y. Q. (2018). A study of ice crystal development in hairtail samples during different freezing processes by cryosectioning versus cryosubstitution method. International Journal of Refrigeration-Revue Internationale Du Froid, 87(3), 39–46. https://doi.org/https://doi.org/10.1016/j.ijrefrig.2017.10.014
- Lucas, T., & Raoult-Wack, A. L. (1998). Immersion chilling and freezing in aqueous refrigerating media: Review and future trends. International Journal of Refrigeration, 21(6), 419–429. https://doi.org/https://doi.org/10.1016/S0140-7007(98)00014-0
- Qian, P., Zhang, Y. Q., Shen, Q., Ren, L. P., Jin, R. Y., Xue, J., Yao, H. Z., & Dai, Z. Y. (2018). Effect of cryogenic immersion freezing on quality changes of vacuum-packed bighead carp (Aristichthys nobilis) during frozen storage. Journal of Food Processing and Preservation, 42(6), 7. https://doi.org/https://doi.org/10.1111/jfpp.13640
- Shi, L., Yang, T., Xiong, G. Q., Li, X., Wang, X. H., Ding, A. Z., Qiao, Y., Wu, W. J., Liao, L., & Wang, L. (2018). Influence of frozen storage temperature on the microstructures and physicochemical properties of pre-frozen perch (Micropterus salmoides). Lwt-Food Science and Technology, 92(6), 471–476. https://doi.org/https://doi.org/10.1016/j.lwt.2018.02.063
- Soares, N. M. F., Oliveira, M. S. G., & Vicente, A. A. (2015). Effects of glazing and chitosan-based coating application on frozen salmon preservation during six-month storage in industrial freezing chambers. LWT - Food Science and Technology, 61(2), 524–531. https://doi.org/https://doi.org/10.1016/j.lwt.2014.12.009
- Subramanian, T. A. (2007). Effect of processing on bacterial population of cuttle fish and crab and determination of bacterial spoilage and rancidity developing on frozen storage. Journal of Food Processing and Preservation, 31(1), 13–31. https://doi.org/https://doi.org/10.1111/j.1745-4549.2007.00101.x
- Sun, Q. X., Sun, F. D., Xia, X. F., Xu, H. H., & Kong, B. H. (2019). The comparison of ultrasound-assisted immersion freezing, air freezing and immersion freezing on the muscle quality and physicochemical properties of common carp (Cyprinus carpio) during freezing storage. Ultrasonics Sonochemistry, 51(2), 281–291. https://doi.org/https://doi.org/10.1016/j.ultsonch.2018.10.006
- Tello Alonso, H. A., Peralta, J. M., Rubiolo, A. C., & Zorrilla, S. E. (2011). Prediction of the freezing point of multicomponent liquid refrigerant solutions. Journal of Food Engineering, 104(1), 143–148. https://doi.org/https://doi.org/10.1016/j.jfoodeng.2010.12.006
- Tsironi, T., Dermesonlouoglou, E., Giannakourou, M., & Taoukis, P. (2009). Shelf life modelling of frozen shrimp at variable temperature conditions. Lwt-Food Science and Technology, 42(2), 664–671. https://doi.org/https://doi.org/10.1016/j.lwt.2008.07.010
- Wang, Y., Wang, X. F., Wang, Z. P., Li, X. F., Li, C., & Xu, B. C. (2020). Effects of different freezing methods on the quality of conditioned beef steaks during storage. Journal of Food Processing and Preservation, 44(7), 11. https://doi.org/https://doi.org/10.1111/jfpp.14496
- Wei, P., Zhu, K., Cao, J., Dong, Y., Li, M., Shen, X., Duan, Z., & Li, C. (2021). The inhibition mechanism of the texture deterioration of tilapia fillets during partial freezing after treatment with polyphenols. Food Chemistry, 335(2), 127647. https://doi.org/https://doi.org/10.1016/j.foodchem.2020.127647
- Wu, X.-F., Zhang, M., Adhikari, B., & Sun, J. (2017). Recent developments in novel freezing and thawing technologies applied to foods. Critical Reviews in Food Science and Nutrition, 57(17), 3620–3631. https://doi.org/https://doi.org/10.1080/10408398.2015.1132670
- Xu, Y. S., song, M., Xia, W. S., & Jiang, Q. X. (2019). Effects of freezing method on water distribution, microstructure, and taste active compounds of frozen channel catfish (Ictalurus punctatus). Journal of Food Process Engineering, 42(1), 9. https://doi.org/https://doi.org/10.1111/jfpe.12937
- Yanco, G. (1981). Phase transitions of water in some products of plant origin at low and superlow temperatures. Advances in Food Research, 27(1), 297–361. https://doi.org/https://doi.org/10.1016/S0065-2628(08)60301-8
- Yang, F., Jing, D. T., Diao, Y. D., Yu, D. W., Gao, P., Xia, W. S., Jiang, Q. X., Xu, Y. S., Yu, P. P., & Zhan, X. B. (2020). Effect of immersion freezing with edible solution on freezing efficiency and physical properties of obscure pufferfish (Takifugu Obscurus) fillets. Lwt-Food Science and Technology, 118(8), 108762. https://doi.org/https://doi.org/10.1016/j.lwt.2019.108762
- Yang, Z. X., Liu, S. S., Sun, Z. P., Xu, W. H., Ji, C. F., Liang, H. P., Li, S. J., Yu, C. X., & Lin, X. P. (2020). Microbial sucession and the changes of flavor and aroma in Chouguiyu, a traditional Chinese fermented fish. Food Bioscience, 37(5), 100725. https://doi.org/https://doi.org/10.1016/j.fbio.2020.100725
- Yasemi, M. (2017). Prevention of denaturation of freshwater crayfish muscle subjected to different freeze-thaw cycles by gelatin hydrolysate. Food Chemistry, 243(21), 199–204. https://doi.org/https://doi.org/10.1016/j.foodchem.2017.04.183
- Yu, L. X., Jiang, Q. X., Yu, D. W., Xu, Y. S., Gao, P., & Xia, W. S. (2018). Quality of giant freshwater prawn (Macrobrachium rosenbergii) during the storage at-18 degrees C as affected by different methods of freezing. International Journal of Food Properties, 21(1), 2100–2109. https://doi.org/https://doi.org/10.1080/10942912.2018.1484760
- Zhang, B., Deng, S.-G., & Wang, Q. (2015). Chemical changes related to loss of quality in pacific white shrimp (Litopenaeus vannamei) during chilled storage under slurry ice conditions. Journal of Food Processing and Preservation, 39(6), 2507–2515. https://doi.org/https://doi.org/10.1111/jfpp.12500
- Zhang, M. C., Xia, X. F., Liu, Q., Chen, Q., & Kong, B. H. (2019). Changes in microstructure, quality and water distribution of porcine longissimus muscles subjected to ultrasound-assisted immersion freezing during frozen storage. Meat Science, 151, 24–32. https://doi.org/https://doi.org/10.1016/j.meatsci.2019.01.002
- Zhao, J., Lv, W. J., Wang, J. L., Li, J. R., Liu, X. X., & Zhu, J. L. (2013). Effects of tea polyphenols on the post-mortem integrity of large yellow croaker (Pseudosciaena crocea) fillet proteins. Food Chemistry, 141(3), 2666–2674. https://doi.org/https://doi.org/10.1016/j.foodchem.2013.04.126