Abstract
Thermal properties of tofu were determined in the temperature range of 5–80°C (10–105°C for specific heat) and the moisture content range of 0.3–0.7 (wb). Thermal conductivity (k: 0.24–0.43 W m−1 K−1) and thermal diffusivity (α: 7.6 × 10−8–1.15 × 10−7 m2 s−1) were measured simultaneously using dual probe method, and specific heat (C p : 2.52–3.69 kJ kg−1 K−1) with modulated differential scanning calorimetry. Good agreement was observed between perpendicular model and the thermal conductivity data, but not with parallel model. Simple empirical models were also developed as a function of the moisture content and temperature of the tofu.
Introduction
Thermal properties of a product depend mostly on its composition and temperature. For porous products, such as bakery products, heat transfer by evaporation and condensation of water often affects the thermal properties as well.Citation[1] Knowledge of thermal properties is important for mathematical modeling and simulation of heat and moisture transport. With the advent of high‐speed computers and inexpensive memory, thermo‐physical properties of target products can be treated as composition/temperature dependent variables instead of the average values over the whole process.
Tofu (soybean curd) is a versatile protein‐rich food and has traditionally been used as a source of protein or meat substitute in Eastern diets. The nutritional value (65% of net protein utilization) and functional properties (anti‐cancer, brain activity elevation) of tofu attract people across the world.Citation[2] Tofu industry is continuously growing from domestic scale to mass scale, and various thermal processing, such as, drying, baking, frying is being applied for better organoleptic properties and microbial safety. During the cooking process, due to heat and mass transfer, temperature and moisture content change. Thus, in order to control the process and design new equipment, the thermal properties of tofu should be known for various ranges of moisture content and temperature. Little work has been published on the thermal properties of the tofu.
Kong et al.Citation[3] determined thermal diffusivity of the tofu (fresh and frozen) at different moisture contents (mass fraction of water 1–0.65, initial moisture content: 0.8 wb) and at −11 to 3°C using 1‐dimensional unsteady heat transfer through the product. They confirmed that the tofu could be considered homogenous in terms of heat transfer, and effective thermal diffusivity was a function of composition and temperature.
The objectives of this study were to estimate the thermal properties of the tofu, and to develop prediction models of the thermal properties for possible use in the simulation of transport phenomenon during thermal processing of the tofu.
Materials and Methods
Tofu
Commercial tofu (extra firm variety, 350 g packets, Toronto based manufacturer) were obtained. For uniform quality, outer layer of tofu (∼0.4 cm) was removed using a sharp knife due to its possible unique hardness caused from mechanical pressure during its processing. Cylindrical samples (D: 5.7 cm, H: 3.5 cm) were cut using a sharp cylindrical cutter for thermal conductivity and diffusivity measurement. Initial chemical composition of the samples were 70.3% water, 15.6% protein, 10.7% fat, 2.0% carbohydrates, 1.1% fiber, and 0.29% ash. Moisture content was determined by drying method.Citation[4] Other compositions were from chemical analysis provided by the manufacturer.
Dehydration
For five levels of moisture content in the range of 0.3–0.7 (wb), fresh tofu was dried in a convection oven (Fisher Isotemp oven® deluxe model/forced draft, Fisher Scientific Inc., Nepean, Ontario, Canada) at 70°C (5% relative humidity) for 0, 2, 8.3, 25, and 46.6 h. During drying, samples were placed on a metal screen support for the exposure of bottom surface to hot flowing air. For faster drying, 90°C air temperature was used in preliminary tests, however, it caused internal shrinkage which lead to inner structure breakage. Thermal deterioration effect if any affected the thermal properties of tofu, which is needed as the tofu is inherently a cooked product through its preparation, and the objective of this study was to evaluate the thermal properties of tofu for various thermal processes. After the completion of drying, samples were kept in a refrigerator (∼3°C) for one week for their internal moisture equilibrium.
Specific Heat
Sample specific heats were determined using a modulated differential scanning calorimeter, (MDSC 2920, TA Instruments Inc., New Castle, DE) with a nitrogen cooling system. Temperature modulation (sinusoidal oscillation) of MDSC separates total heat flow [EquationEq. 1] into its reversing [specific heat related, MC p (dT/dt)] and non‐reversing [kinetic, f (t,T )] components. The f (t,T ) denotes specific response that is kinetically hindered and takes a measurable amount of time to come to equilibrium when the temperature is changed, such as in glass transition, crystallisation, melting, chemical reactions, etc. For its calculation, the amplitudes of modulations in heat flow and heating rate, phase angle are required and they are measurable by the MDSC.
Specific heat was also estimated from measured thermal conductivity, thermal diffusivity, and bulk density by applying the following relationship.
Thermal Conductivity and Thermal Diffusivity
A commercial thermal property‐meter (Thermolink TL‐1, Devices Inc., Pullman, WA) was used to measure thermal conductivity and thermal diffusivity of the tofu. The instrument was designed based on line heat source probe and additional thermocouple probe method. The merit of this method is the simultaneous measurement of both properties. The dual probes (1.0 mm o.d. and 30 mm long) consisted of a constantan heater wire and a chromel–constantan E‐type thermocouple (TLS‐1, Decagon Devices Inc., Pullman, WA). The probes were spaced 6 mm apart. Their location was in the following ranges suggested by Nix et al.Citation[6]
The rate of temperature increase in the cylindrical heater is directly related to the diffusivity as shown in the following equations:
For test at T < 10°C, tofu samples were directly transferred from the refrigerator into a insulation box. The probe was embedded inside the box cover () and then the probe was fully inserted into the sample placed in the box with the linear movement through nuts to avoid breakage of the sample. For maintaining the probe spacing, a probe guide was placed on the end of the probe needles and then the needles were gently inserted all the way into the tofu samples. After each test, the spacing was verified by cutting inner part of the tofu sample and measuring the distance between two needle marks. A few wrong insertions made spacing errors, and the measured data were discarded for those experiments. The material of bolts and nuts was steel. Thermal conductivities of the insulation material (extruded polystyrene form) and the gasket (formed rubber) were 0.026 and 0.034 W m−1 K −1 at 300 K, respectively. The samples were thermally equilibrated for at least 40 min. For higher temperatures, samples in the hermetic plastic bag were heated in water bath for ∼40 min, then samples were immediately moved from the platic bag into the sample holder, which was water proof. The samples were thermally equilibrated for 1.5–2 h based on preliminary test results. After each test, moisture content of the portion between two probes was determined. This moisture content was used in the regression analysis. At the beginning of this study, microwave heating was tried for the sample heating. In spite of the application of low power/long time, considerable pores were formed in the sample during heating. Water bath heating did not cause this problem. A sample diameter of 57 mm was selected to avoid edge effect, based on Campbell et al.Citation[7] as follows:
Data Analysis and Modeling
All experiments for each treatment were conducted in triplicate. A factorial experimental design was used. For C p test, each different sample was used for three determinations; for k and α test, one same sample for three determinations. Statistical analysis was conducted using PROC REGCitation[9] to develop regression models for the thermal properties of the tofu. The step‐wise regression procedure was used for selecting variables to be included in the model. The variables considered in the procedure were m, T, m 2 and T 2 and all interaction terms. Density was not included in the model to avoid multicollinearity among the variables since density often can be related to moisture content. To select the best‐fitting model, two criteria were considered, the correlation coefficient R 2 and the F value of the model.
Results and Discussions
Specific Heat
Specific heat of the tofu containing moisture content of 0.33–0.68 (wb) was 2.52–3.69 kJ kg−1 K−1 for temperature range of 10–105°C. Specific heat of tofu increased with moisture content and temperature (). Calculated C
p
values from mass fraction model (∑ X
i
w
C
pi
) were compared with corresponding measured values. First, six major tofu components (water, protein, carbohydrate, fat, fiber, and ash) were considered; and then only two components were taken (water and total dry matter including fat). The C
p
of dry matter was determined by thermal scanning of a fully dried tofu using MDSC. For other six components, literature dataCitation[10] were used. Visual comparison showed a good agreement with experimental data (). The RMS (root mean square deviation; , where, N = number of C
p
values) between predictive values (C
pcal
) from two models and experimental data (C
pexp
) were 0.0987 and 0.103, respectively () indicating the change at one decimal place. An empirical model as a function of moisture content and temperature was developed using stepwise method of PROC REG.Citation[9] A summary of the stepwise selection of variables is given in . The suitable model is:
Figure 3. Specific heat calculated from an empirical model [EquationEq. 9] as a function of moisture content and temperature of the tofu.
![Figure 3. Specific heat calculated from an empirical model [EquationEq. 9] as a function of moisture content and temperature of the tofu.](/cms/asset/3f61de4e-ea60-4d73-b699-ce12dbcd9514/ljfp_a_10345089_o_f0003.gif)
Table 1a. Specific heat (kJ kg−1 K−1) data of the tofu at different moisture contents and temperatures
Table 1b. Thermal conductivity and thermal diffusivity data of the tofu at different moisture contents and temperatures
Table 2. Thermal property models and root mean square errors in comparison to experimental data
Table 3. Summary of the stepwise selection of variables in the empirical model of specific heat of the tofu
Specific heat was also calculated from measured thermal conductivity, diffusivity, and density. The apparent density of tofu was 1053–1251 kg m−3 for the moisture content range of 0.34–0.73 (wb). Linear relationship was observed between density and moisture content:
The residuals vs. predicted ρ and the moisture content term in the model were normally distributed. For shrinkage modeling of a solid material, the following assumption is often applied. Thermal expansion of a sample is negligible compared to shrinkage due to moisture migration for a non‐porus solid material. Good agreements were reported between the calculated data from this assumption and experimental data.Citation[11],Citation[12] Therefore, temperature effect was neglected in the correlation. The calculated specific heat was slightly lower (∼1.7%) than those measured with MDSC, however, the difference was not significant (p = 0.17) when using t‐test (DF = 132). Direct measurement using MDSC should be more accurate as calculated C p already has inherent deviation from experimental errors of three other thermophysical properties.
Thermal Conductivity
Thermal conductivity of the tofu was 0.24–0.42 W m−1 K−1 for moisture content range of 0.34–0.72 and temperature range of 6–74.2°C (). Yano et al.Citation[13] reported thermal conductivity of frozen and fresh tofu (physical characteristics, such as variety, moisture content, temperature, and density of tofu were not specified) as 0.49 and 0.30 W m−1 K −1, respectively. Thermal conductivity of the tofu increased with increase in temperature and moisture content. Predicted values from the following structural models were compared with experimental data ().
Figure 4. Comparison between experimental and calculated thermal conductivity from structural models and empirical model.
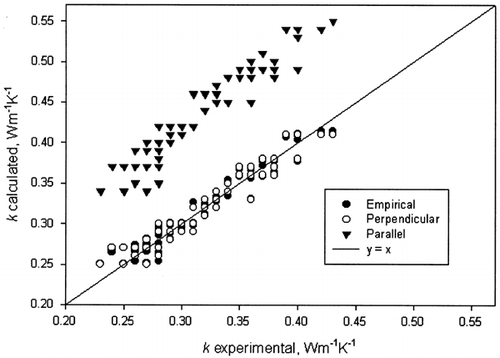
Predicted values from the parallel model did not match well with experimental values, and indicating a RMS of 0.119. The perpendicular model provided good predictions with a RMS of 0.0118. This was consistent with the observation of Yano et al.Citation[13] Statistical analysis showed that the effects of moisture content (m 2) and interaction between moisture content and temperature (m × T ) were significant ( p < 0.0001) (). The model is:
Figure 5. Thermal conductivity calculated from an empirical model [ EquationEq.13] as a function of moisture content and temperature of the tofu.
![Figure 5. Thermal conductivity calculated from an empirical model [ EquationEq.13] as a function of moisture content and temperature of the tofu.](/cms/asset/089bcc83-2b14-4293-9ca9-8cd75fe7a107/ljfp_a_10345089_o_f0005.gif)
Table 4. Summary of the stepwise selection of variables in the empirical model of thermal conductivity of the tofu
Thermal Diffusivity
For moisture content range of 0.34–0.72 (wb) and temperature range of 6–74.2°C, the thermal diffusivity of the tofu were 7.6 × 10−8–1.15 × 10−7 m2 s−1. These values are slightly lower than those (1.1 × 10−7–1.3 × 10−7 m2 s−1) of the tofu with moisture content range of 0.52–0.8 (wb) and temperature range of 0–3°C reported by Kong et al.Citation[3]
Martens'regression modelCitation[14] [ EquationEq.14] based on 246 published values of thermal diffusivity of many food products were compared with experimental data.
Figure 6. Comparison between experimental and calculated thermal diffusivity from Martens' and empirical model.
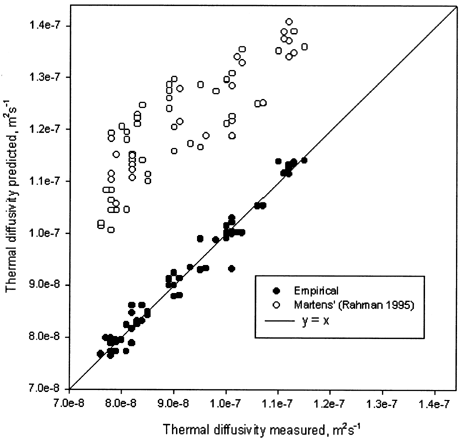
Figure 7. Thermal diffusivity calculated from an empirical model [EquationEq. 15] as a function of moisture content and temperature of the tofu.
![Figure 7. Thermal diffusivity calculated from an empirical model [EquationEq. 15] as a function of moisture content and temperature of the tofu.](/cms/asset/f80e0c2a-4608-4010-b176-340729b50881/ljfp_a_10345089_o_f0007.gif)
Table 5. Summary of the stepwise selection of variables in the empirical model of thermal diffusivity of the tofu
Conclusions
Thermal properties of tofu were measured for various ranges of moisture content and temperature. Predicted properties from mass fraction model and perpendicular model showed good agreement with experimental data of specific heat and thermal conductivity, respectively. Empirical regression models were developed as a function of moisture content and temperature.
Acknowledgments
The first author thanks Fond FCAR (Le Fonds pour la Formation de Chercheurs et l'Aide à la Recherche) for providing post‐doctoral scholarship for this research. The authors gratefully acknowledge NSERC (Natural Science and Engineering Research Council of Canada) for research funding.
Nomenclature | ||
a | = |
Agar content in weight percentage (g agar/100 g water) |
C p | = |
Specific heat, kJ kg−1 K−1 |
k | = |
Thermal conductivity, W m−1 K−1 |
M | = |
Mass, kg |
m | = |
Wet basis moisture content in mass ratio |
Q | = |
Power dissipated by probe heater, J m−1 or heat provided to the sample, J |
r d | = |
Distance in radial direction, m |
r s | = |
Sample radius, m |
T | = |
Temperature, °C |
t | = |
Time, s |
X i w , X i v | = |
Mass fraction, volume fraction |
α | = |
Thermal diffusivity, m2 s −1 |
ρ | = |
Apparent density, kg m−3 |
Subscript | ||
cal | = |
calculated |
exp | = |
experimental |
i | = |
unit component |
References
- Baik , O. D. , Marcotte , M. , Sablani , S. S. and Castaigne , F. 2001 . Thermal and physical properties of bakery products . Crit. Rev. Food Sci. Nutr. , 41 ( 5 ) : 321 – 352 .
- Shurtleff , W. and Aoyagi , A. 1983 . Book of Tofu 336 Berkeley , CA : Ten Speed Press .
- Kong , J. Y. , Miyawaki , O. and Yano , T. 1980 . Effective thermal diffusivities of some protein gels . Agric. Biol. Chem. , 44 ( 8 ) : 1905 – 1910 .
- 1990 . “ AOAC ” . In Official Methods of Analysis Washington DC : Association of Official Analytical Chemists .
- 1995 . “ TA Instruments. Modulated DSCTM Option Appendix E ” . In DSC2910, Differential Scanning Calorimeter Operator Manual New Castle : TA Instruments .
- Nix , G. H. , Lowery , G. W. , Vachon , R. I. and Tanger , G. E. 1967 . “ Direct determination of thermal diffusivity and conductivity with a refined line source technique ” . In Progress in Astronautics Edited by: Heller , G. R. Vol. 20 , 865 – 878 . New York : Academic Press .
- Campbell , G. S. , Calissendorff , C. and Williams , J. H. 1991 . Probe for measuring soil specific heat using a heat‐pulse method . Soil Sci. Soc. Am. J. , 55 ( 1 ) : 291 – 293 .
- Kreith , F. and Bohn , M. S. 1993 . Principles of Heat Transfer A14 St‐Paul , MN : West Publishing Co. . 5th Ed.
- 1996 . “ SAS Institute, Inc ” . In PC‐SAS User's Guide, Version 6 Cary , NC : SAS Institute, Inc. .
- Choi , Y. and Okos , M. R. 1986 . “ Effects of temperature and composition on thermal properties of foods ” . In Food Engineering and Process Alications Vol. 1 , 83 London : Elsevier .
- Bowser , T. J. and Wilhelm , L. R. 1995 . Modeling simultaneous shrinkage and heat and mass transfer of a thin, nonporous film during drying . J. Food Sci. , 60 : 753 – 757 .
- Simal , S. , Rossello , C. , Berna , A. and Mulet , A. 1998 . Drying of shrinking cylinder‐shaped bodies . J. Food Eng. , 37 : 423 – 435 .
- Yano , T. , Kong , J. Y. , Miyawaki , O. and Nakamura , K. 1978 . Prediction of thermal conductivity of tofu and meats . Int. Cong. Food Sci. Tech.‐Abs. , : 115
- Rahman , S. 1995 . Food Properties Handbook 314 – 319 . 377 Boca Raton , FL : CRC Press, Inc. .
- Murakami , E. G. and Okos , M. R. 1989 . “ Measurement and prediction of thermal properties of foods ” . In Food Properties and Computer‐aided Engineering of Food Processing Systems Edited by: Singh , R. P. and Medina , A. G. 3 – 48 . Norwell , MA : Kluwer Academic Publishers .
- Rask , C. 1989 . Thermal properties of dough and bakery products: a review of published data . J. Food Eng. , 9 : 167 – 193 .