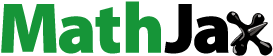
ABSTRACT
Edible cassava leaves (Manihot esculenta) were consumed as vegetables for their bioactive compounds, however the effect of harvesting time on these bioactive compounds and their antidiabetic and anti-obesity inhibitory activities were scarcely reported in the literature. Phenolics from edible leaves harvested at different times were assessed using HPLC-DAD, and their antidiabetic and anti-obesity inhibitory activities were investigated before and after in vitro gastrointestinal digestion. The results showed a significant (P < .05) effect of harvesting time on phenolic contents. Bound phenolics showed higher lipase inhibitory activity than their free fractions. However, phenolic contents decreased after in vitro digestion, while they showed high antidiabetic and anti-obesity inhibitory activities. The present study demonstrated that edible leaves harvested at different times are rich in flavonoids, stilbenes, tannins and saponins. These bioactive compounds are highly potent against α-amylase, α-glucosidase and lipase before and after in vitro gastrointestinal digestion. Therefore, we recommend edible cassava leaves for important biological active compounds for the prevention of obesity and type 2 diabetes.
Introduction
Non-communicable diseases such as obesity, Alzheimer's, diabetes, stroke or cardiovascular disease are the most important diseases affecting a significant section of world population in the different regions of the world. These diseases have become global health problems with a high prevalence.[Citation1] Diabetes affects 415 million people worldwide and this number is projected to rise to 642 million by 2040.[Citation2] It is demonstrated that the bioactive compounds in plants have the potential to reverse or prevent obesity, cardiovascular disease and diabetes.[Citation3,Citation4] In addition, dietary polyphenols contribute to lower glycemic indices by inhibiting intestinal glucose transport proteins thus reduce the risk of type 2 diabetes.[Citation5] Furthermore, some evidences demonstrate that eating phenolic-rich foods, particularly flavonoids, is inversely correlated with the prevalence of cardiovascular diseases and type 2 diabetes.[Citation6,Citation7] Flavonoids are well known as antioxidants, are used in obesity and diabetes type 2 prevention, to suppress cholesterol levels, as anti-inflammatory, antiviral, anti-cardiovascular disease, anticancer and antitumor.[Citation8] Flavonoids may reduce insulin resistance and lipid accumulation by various ways and promote glucose and lipid metabolism.[Citation9] For example, quercetin can interact with various molecular targets, such as small intestine, pancreas, adipose tissue and liver, hence regulating homeostasis of human body glucose[Citation10]. The inhibitory activity may also be due to the possible dynamic-binding mechanism of the flavonoids with α-glucosidase and α-amylase.
Cassava leaves are used to treat headaches, fevers, diarrhea, rheumatic diseases, inflammation and gastritis for many centuries because of their antioxidant properties.[Citation11,Citation12] Consumption of cassava leaves rich in phytochemicals, such as phenolic acids, saponins, stilbenes, carotenoids and flavonoids, may act to prevent chronic degenerative diseases as well as other non-communicable diseases. Many studies have proven that cassava leaves apart for being rich in nutritional composition, they have good concentration of these phenolics compounds and higher antioxidant capacity.[Citation13–15] Several other studies revealed that polyphenols in vegetables exist both in bound and free form,[Citation16] and their bound fraction can be released by the action of microbial flora and exert their higher bioactivity.[Citation16] Furthermore, bound polyphenols may be released continuously in a slow manner through the gastrointestinal tract and are released abundantly during bacterial fermentation, which can improve their bioaccessibility and bioavailability,[Citation17] hence they can exert high bioactivity on tissues and cells for a long time than free phenolics. It was reported also that bound phenolics are better antioxidant and antitumor agents compared to free phenolics.[Citation18] It is well known that polyphenols composition and antioxidant activity of vegetables are largely affected by plant maturity, seasonal variation and environmental stress.[Citation19] Thus, bound polyphenolics content ignored may be underestimated the biological activities in cassava leaves. Edible cassava leaf is harvested at any time and season for nutritional and health purposes. The levels of bound, free and bioaccessible polyphenols may be affected by the growing seasons, harvesting time, as well as genetic factors, hence, polyphenols and biological properties.
To the best of our knowledge, the variation of flavonoids, stilbenes, saponins and tannins of cassava leaves harvested at different harvesting times, as well as their antidiabetic and anti-obesity inhibitory activities are not reported. Therefore, the purpose of the study was to investigate for these phenolics contents in bound, free and bioaccessible fractions of edible cassava leaves and assessed their antidiabetic (α-amylase and α-glucosidase) and anti-obesity (lipase) inhibitory activities.
Materials and methods
Experimental design
The method described by IITA[Citation20] was used for the experimental design. The cassava varieties improved named TMS92/0326, TMS96/1414 and IRAD4115 obtained from IITA (International Institute of Tropical Agriculture, Ibadan, Nigeria) and IRAD (Institute of Agricultural Research for Development, Ngaoundéré, Cameroon), respectively, and two local varieties named AD and EN were used in the experimental field. During the growing season, the average of precipitations were 1200 mm and 1100 mm for 2014/2015 and 2015/2016, respectively. The temperature varied between 20°C and 45°C during the two growing seasons. The experimental field was located at 10°54ʹ0.0” north latitude and 14°12ʹ0.0” east longitude. Cassava plants (M. esculenta) were grown in an experimental open field under natural conditions in Tokombéré Sub-division from Far North region of Cameroon. Fertilizers and pesticides were not applied during the experiment. The experimental field is conducted in completely randomized blocks with four repetitions of five plots separated by three 2 m rows. Each plot contained 25 plants. Edible cassava leaves from the top (third young) were sampled at different growing stages in 3-month intervals up to 15 months after planting (MAP). During each sampling time, the inner line of plants of each variety from each plot was considered. The leaves of 25 plants were sampled from each cassava variety during the experiment. In addition, cassava leaves were harvested both in dry and wet seasons.
Samples preparation
Edible cassava leaves were washed, cut into pieces and the oven dried at a temperature of 60°C for 24 h (UN75 Memmert Loading-Models 30–750, Federal Republic of Germany). The leaves were then reduced to a powder manually using a mortar and a pestle. The ground material was sieved through a 200 μm mesh and stored tightly in opaque polystyrene bag (Handgards®, Texas, USA) prior to analysis.
Chemicals and reagents
All phenolic standards such as rutin (purity >98%), catechin (purity >98%), kaempferol (purity >98%), resveratrol (purity >98%), diosgenin (purity >93%), tannic acid (purity >98%), quercetin (purity >95%), acarbose (purity >95%), and orlistat (purity >98%), and different enzymes and substrates, such as α-glucosidase (purity >97%), hog pancreatic α-amylase (purity >95%), porcine pancreatic lipase (purity >98%), p-Nitrophenyl butyrate (pNPB) (purity >99%), and p-Nitrophenol palmitate (p-NPP) (purity >98%), 3,5-Dinitrosalicylic acid (DNS) (purity >98%) were obtained through Sigma-Aldrich (Mumbai, India). Other chemicals (purity >99%) and reagents (purity >98%) used were of analytical grade purchased from the Sisco Research Laboratory (Bengaluru, India)
Free and bound polyphenolic extraction
Free and bound phenolic compounds were extracted following the method described by Chen et al.[Citation21] with minor changes as described by Laya and Koubala.[Citation22]
In vitro gastrointestinal digestion
The method described by Ydjedd et al.[Citation23] with some modifications was used in the present study. Briefly, the simulated solution was prepared in a phosphate buffer (1 M, pH 6.0). The digestion started with the introduction of 1.5 mL of simulated salivary solution to each sample (500 mg) containing 5 mg of porcine α-amylase (1000 U/mg). The pH of the mixture was adjusted to 7 before stirring for 10 min in a shaking water bath at 37°C for 90 rpm. The next step was to add 1.5 mL of the gastric digestion stimulating solution (10 mg/mL) consisting of pepsin (1:3000) initially diluted in HCl (0.1 N). The pH was adjusted to 2 with HCl (6 M) and the mixture was incubated at 37°C in a shaking water bath (90 rpm) for 2 h. To simulate intestinal digestion, 2 mL of the intestinal solution (10 mg/mL) of pancreatin (100 U/mg) and 4 mg/mL of bile salts (100 U/mg) were added, respectively. The pH was adjusted to 7 with NaOH (1 M), and the mixture was incubated for 3 h in a shaking water bath at a speed of 90 rpm. The enzymatic reaction was stopped by adding 1 mL of methanol (HPLC grade). The supernatant was recovered and 1 mL of methanol (HPLC grade) was added and centrifuged at 4°C at 10,000 rpm for 10 min. After filtration, the solvent was then evaporated and the residue was dissolved in methanol (HPLC grade) and filtered with the 0.22 μm membrane (polypropylene, USA) and kept at −20°C until the analysis.
Flavonoids in bound, free and bioaccessible polyphenols
Free, bound and bioaccessible flavonoids content were determined as described by Xiong et al.[Citation24] Absorbance was recorded at 510 nm using a multimode microplate reader (Tecan SPARK 10 M, V1.2.20, Austria). The quercetin was used as a standard.
Tannins in bound, free and bioaccessible polyphenols
Tannins content were determined using the vanillin reagent according to Gaytan-Martínez et al.[Citation25] The absorbance was taken at 500 nm in a multimode reader (Tecan SPARK 10 M, V1.2.20). The tannic acid was used as a standard.
Condition for HPLC-DAD analysis of polyphenols
The HPLC system (Hitachi Elite LaChrom System, Hitachi High Technologies Japan, Tokyo, Japan) equipped with a Shodex C18-120-5 4E column, DAD detector and auto sampler, was used for quantification of phenolics. The C18 column (4.6 × 250 mm, 5 μm) (Supelco. Co) was used for separation of phenolics. System control and data acquisition were performed by Empower 3 Software (2010) (Corporation, Kyoto, Japan). Free and bound phenolics were filtered with RC 0.45 µm (Phenex, USA) and filled in HPLC vials (Waters 1.5 ml, USA). The mobile phases consisted of: (A) Milli-Q water containing 1% acetic acid (v/v) and (B) methanol containing 1% acetic acid (v/v). The column temperature was maintained at 30°C and 20 μL of each sample was automatically injected. The samples as well as standards were injected in triplicate. Elution was carried out at a flow rate of 1 mL/min. The linear gradient was formed as follows: 0–7 min (15% B), 7–30 min (50% B), 30–50 min (100% B), 50–60 min (0% B), 60–70 min (0% B). Phenolics were monitored at 280, 320 and 360 nm. Peak of each phenolic was identified with authentic standard by comparing the retention time, and their quantification was done using standard curves established at eight (08) different levels over a concentration ranged of 5 to 100 μg/mL) for individual standard. Chromatograms of standards (Figure S1) of some samples (Figure S2) are presented in supplementary data.
α-glucosidase inhibitory activity of polyphenols
The α-glucosidase inhibitory activity was measured according to the method described by Zhang et al.[Citation26] with some modifications. Briefly, 20 µL of sample or standard and 40 µL of pNPG (4 mM 4-nitrophenyl-α-D-glucopyranoside) solution were filled in microplates well. Thereafter, the mixture was preincubated in a water bath at 37°C for 15 min. Then, 20 µL of the enzyme (10 units/mL) prepared in PBS (0.1 M, pH 6.8) was added in each well and incubated in water bath at 37°C for 40 min. The reaction was stopped by adding 100 µL Na2CO3 (1 M) and cooled at room temperature. Finally, the p-Nitrophenol released from pNPG was measured at 405 nm using a multimode reader (Tecan SPARK 10 M, V1.2.20, Austria). Before taking the absorbance at 405 nm, 20 µL of milli-Q-water was added into each well. To eliminate the background absorbance produced by cassava leaf extracts, a control without enzyme was considered. Acarbose was used as a positive control.
where A sample is the absorbance of the mixture of sample, pNPG, enzyme solution; A blank is the absorbance of the mixture of sample, pNPG solution without enzyme; A control is the absorbance of the mixture of buffer, pNPG and without enzyme; A test is the absorbance of the mixture of buffer instead sample, pNPG and enzyme solution.
α-amylase inhibitory activity of bound, free and bioaccessible polyphenols
The α-Amylase inhibitory activity was measured according to the method of Zhang et al.[Citation26] modified by Tan et al.[Citation27] with some modifications. For this assay, 20 µL of sample or standard and 80 µL 1% starch were filled in microplates well 96. Thereafter, the mixture was incubated in a water bath at 37°C for 15 min. Then, 20 µL of the enzyme (30 units/mL) prepared in PBS (0.1 M, pH 6.8) was filled in each well before incubating in a water bath at 37°C for 10 min. Twenty microliters of DNS were added into each well and incubated at 95°C for 40 min. Before taking the absorbance at 540 nm, using a multimode reader (Tecan SPARK 10 M, V1.2.20, Austria), 20 µL of milli-Q water was added into each well. To minimize the background absorbance produced by cassava leaf extracts, a control without enzyme was considered.
where A sample is the absorbance of the mixture of sample, starch, enzyme and DNS solution; A blank is the absorbance of the mixture of sample, starch and DNS solution without enzyme; A control is the absorbance of the mixture of buffer, starch, enzyme and DNS solution without enzyme; A test is the absorbance of the mixture of buffer, starch, enzyme and DNS solution.
Lipase inhibitory activity of bound, free and bioaccessible polyphenols
The lipase inhibitory activity was measured based on the method of Zhang et al.[Citation26] modified by Tan et al.[Citation27] with some modifications. Briefly, 20 µL of sample or standard and 40 µL of pNPP (p-Nitrophenol palmitate) solution were filled in microplates well. Thereafter, the mixture was preincubated in a water bath at 37°C for 15 min. Then, 20 µL of the enzymes (10 units/mL) prepared in buffer (0.1 M, pH 6.8) was filled in each well before incubating in water bath at 37°C for 40 min. After cooling at room temperature, absorbance was measured at 410 nm using a multimode reader (Tecan SPARK 10 M, V1.2.20, Austria). To eliminate the background absorbance produced by cassava leaves extracts, a control without enzyme was considered.
where A sample is the absorbance of the mixture of sample, pNPP, enzyme solution; A blank is the absorbance of the mixture of sample, pNPP solution without enzyme; A control is the absorbance of the mixture of buffer (instead of sample) and pNPP without enzyme; A test is the absorbance of the mixture of buffer, pNPP and enzyme solution.
Statistical analysis
All data were analyzed by ANOVA using Statgraphics software (version. 16). Tukey’s post hoc test was used to determine any significant difference between different varieties in the same month and in all harvest times for each variety and significance was accepted at level p < .05. The results were expressed as means ± standard deviation. All experiments were carried out in four repetitions except individual phenolic in triplicate.
Results and discussion
Flavonoid contents (TFC) of edible leaf
There was significant (p < .05) variation in TFC of bound fraction during the harvests except at 6 MAP (). However, throughout harvesting the highest value (1.21 mgQE/g) was shown by IRAD4115 variety at 15 MAP. Meanwhile, the free polyphenols of EN variety exhibited the highest concentration of TFC (4.53 mgQE) at 6 MAP and the differences among varieties were significant (). The highest TFC of free polyphenols could be attributed to dry season with severe water stress in the locality when plants increase non-enzymatic antioxidants to survive; however, the variation among varieties may be due also to the genetic variability in response to this environmental stress. These results are in agreement with Yang et al.[Citation28] who reported a significant increase in flavonoid content during water stress in plants. The lowest concentration of TFC of bound and free fraction were shown by TMS92/0326 (0.14 mgQE/g) at 6MAP and TMS96/1414 (1.09 mgQE/g) varieties at 12MAP, respectively (). These variations occurred during dry and wet seasons were consistent with the results of Bhandari and Kwak,[Citation29] who reported great variations in the amounts of flavonoids in leaves of 12 varieties of broccoli at rainy and dry season. The age of the plants may affect the composition of edible leaves as the plants were stressed by drought. The present study showed that the harvest times had great influence on the concentration of TFC in bound and free fractions of polyphenols in cassava leaves.
Table 1. Total flavonoids and total tannins content of bound and free (undigested) and bioaccessible (simulated digested sample) polyphenols
Table 2. Individual flavonoids content of bound and free fractions (undigested sample)
The bioaccessible TFC of the edible leaves of varieties varied between 0.11 and 0.98 mg/g as presented in . The leaves of the IRAD4115 variety harvested at 15 MAP showed considerable bioaccessibility of TFC. These results could be explained by the influence of the variety, which could have favored the food matrix more accessible to the digestive enzymes to release compounds. However, edible leaves harvested at 6 MAP from AD variety (0.11 mg/g) showed significantly (p < .05) the lowest bioaccessible TFC (). These results may be due to the low solubilization of flavonoids in the leaves harvested at 6 MAP; however, that may be more bioaccessible in the large intestine by the action of microbial flora with the highest bioactivity.[Citation30]
Total tannin contents (TTC) of edible leaf
The TTC varied significantly (p < .05) at different harvesting times. For the bound fraction, the highest value of TTC (3.52 mgTAE/g) was shown by AD variety at 12 MAP (), while the IRAD4115 variety showed the highest concentration (22.24 mg TAE) of TTC for free phenolics at 9 MAP (). It was noted that the highest value of TTC coincided with the dry season in the locality, which indicated that the plant might use the TTC in response to pest attacks. The TMS92/0326 (0.23 mg TAE/g) and TMS96/1414 (3.09 mg TAE/g) varieties showed the lowest values for TTC of bound and free phenolics at 12 MAP and 15 MAP, respectively. The TTC of both bound and free fractions of polyphenols were significantly higher in dry season in most varieties indicating that the stress induced in plants during the dry season increased the polyphenolics in leaves (). The effect of variety on TTC was highly significant (p < .05), however the harvest stages had a high influence on TTC, as early harvest favored TTC in bound fraction, while the 9 MAP was best for TTC in free fraction. These variations of tannins content during seasonal changes were reported by Ossipov et al.[Citation31] in birch leaf; however, they did not mention the different fractions of polyphenols in their study.
The bioaccessibility of TTC varied through the harvest times (). The TTC of the leaves of the TMS96/1414 variety (0.69 mg/g) harvested at 9 MAP was significantly (p < .05) higher compared to others. In fact, the harvest coincides with the beginning of the dry season, this could explain these results of bioaccessibility of tannins. However, the tannins of the same leaves harvested at 6 MAP were poorly bioaccessible (). This means that tannins of leaves harvested at 6 MAP could be less accessible to digestive enzymes to release tannins from the sample matrix. Furthermore, this suggests that the particle size of the tannins could be larger, hence could not promote their good solubilization during gastrointestinal digestion. These observations were in agreement with Alminger et al.,[Citation32] who reported that high molecular weight polyphenols such as tannins can interact strongly with fibers and proteins, which could significantly limit their bioaccessibility.
Individual polyphenols of bound, free and bioaccessible fractions
The highest value of rutin of bound fraction was observed in leaves of EN variety (71.60 mg/10g) at 6MAP, however the TMS92/0326 variety showed the highest rutin content (1024.92 mg/10g) for free fraction at 15MAP () among the varieties. These findings were consistent with the results of Gouvea et al.,[Citation33] who reported the variations with an increase of flavonoids during rainy season in leaves of E. mattogrossensis. These results may be explained by genetic differences of varieties as well as their response to environmental stress. The lowest values for rutin were showed by TMS96/1414 (0.89 mg/10g) and EN (21.46 mg/10g) varieties at 15 MAP in bound and free fraction, respectively, which could be due to the main rainy season during the harvest. The EN variety (94.95 mg/10 g) showed the best concentration of catechin at 12 MAP in bound polyphenols compared to the other varieties. The highest value of catechin of free fraction was shown by IRAD4115 (451.6 mg/10 g) at 12 MAP (). These results suggest that seasonal variation have an impact on catechin concentration in edible leaves. The results were in agreement with the findings of Ernst et al.[Citation34] who reported that catechin concentration was affected by seasonal variation in leaves of 10 plants. Kaempeferol concentration of bound and free fraction showed a significant difference amongst the varieties. The TMS92/0326 variety (37.03 mg/10 g) showed a significant high concentration of kaempferol in bound fraction at 15MAP (). However, for the free polyphenols, the TMS96/1414 variety (285.57 mg/10 g) showed the highest kaempeferol content at the same harvesting time (). The lowest concentration of kaempeferol observed at 12 MAP for bound polyphenols of the IRAD4115 variety and free polyphenols of AD variety at 15MAP coincided with the rainy season. These results were similar with Anttonen et al. (2006)[Citation35] and Yang et al.[Citation28] who observed the kaempferol concentration affected by season and variety of different strawberry fruits and barley, respectively. Quercetin content in bound fraction was the highest in the TMS92/0326 variety (59.28 µg/100g) at 9MAP (). This result showed that during the dry season, quercetin content increase which may be in relation with antioxidants mechanism to overcome water stress. In fact, quercetin plays an active role in defense against high solar radiation in leaves.[Citation36] The IRAD4115 variety (70.91 µg/g) showed the highest quercetin of free fraction at 15 MAP (), which may be related to the plant maturity. The harvest stage influenced significantly the quercetin concentration of bound and free polyphenols. These results were similar to those reported by Bujor et al.[Citation37] who found that quercetin content of V. vitis-idaea leaves was affected by harvesting stages. In addition, Anttonen et al.[Citation35] reported the effects of environment on quercetin concentration. Similarly, seasonal variation is reported to affect the phenolics concentration in plants species.[Citation33,Citation34] Resveratrol was not detected in bound fraction of EN and AD varieties at 15MAP and in EN variety at 6MAP (). However, the bound fraction of IRAD4115 variety showed the highest value (11.59 µg/g) of resveratrol at 15 MAP, while the free polyphenols of AD variety had the highest concentration (5679.12 µg/g) of resveratrol at 9 MAP. The bound polyphenols of AD variety (0.09 µg/g) at 9MAP and free fraction of the TMS96/1414 variety (0.91 µg/g) at 6MAP exhibited the lowest value of resveratrol (). This result was similar to those reported that the variety influenced the resveratrol concentration similar to barley varieties.[Citation28] The diosgenin content of bound and free fractions extracts varied significantly among varieties during different harvest stages (). However, the highest concentration in the bound fraction was shown by IRAD4115 variety (2.45 g/g) at 12 MAP, while the TMS96/1414 variety (6.95 µg/g) showed the best concentration of diosgenin in free polyphenols at the same harvest stage (). The lowest diosgenin concentration was observed at 9MAP in EN variety (0.01 µg/g) for bound fraction, and IRAD4115 variety (0.02 µg/g) of free fraction (). These differences may be due to the varietal variation as well as plant developmental stages. There was a significant variation among varieties in Tannic acid content during harvests however, the highest concentration of tannic acids was showed by TMS92/0326 variety (580.43 mg/100 g for bound fraction at 6 MAP, and 404.31 mg/g for free fraction at 15 MAP) (). These variations of concentration of tannic acids may be due to the varietal genetics. However, these results are consistent with the reported results of Yang et al.[Citation28] for bound and free fractions of barley varieties.
Table 3. Individual stilbenes, saponins and tannins content of bound and free polyphenols (undigested sample)
The bioaccessible rutin content of leaves of the EN variety at 6MAP was significantly higher with a value of 2798.49 μg/g followed by those of the TMS96/1414 variety (1558.55 μg/g) during the same harvest (), while the leaves of the AD variety harvested at 9MAP showed significantly the lowest value (317.03 μg/g). These results showed the impact of leaf harvest time on the bioaccessibility of rutin. The catechin bioaccessible of leaves of the EN variety harvested at 6MAP were significantly rich with a value of 45.06 μg/g, however at 9MAP, the leaves of the same variety had the lowest value (0.54 μg/g) (). These results could be justified by the varietal ability to react toward environmental changes and the initial concentration of the compounds before in vitro digestion. The bioaccessible kaempferol content varied also significantly from one variety to another during different harvests, and the values varied between 0.64 μg/g for TMS92/0326 at 12MAP and 20.82 μg/g for AD at 15 MAP (). For the bioaccessible quercetin content, the values varied between 0.25 and 6.32 μg/g for the AD and EN varieties at 6MAP, respectively (). These results showed that flavanols, particularly rutin, are more bioaccessible than flavonols. This result is in agreement with Khanam et al.[Citation38] who reported rutin as the most abundant of bioaccessible flavonoids of leafy vegetables. In fact, rutin is in the form of glycosides that resists acid hydrolysis of the stomach and therefore reaches the small intestine. Also, the fact that flavanols are poorly bioaccessible could be attributed to the affinity of enzymes for the cleavage of flavonoids and their glycosides or loss during gastrointestinal digestion.[Citation39] It has been reported that some polyphenols and flavonoids may be less stable and undergo partial or complete degradation during transition of the alkaline and acidic medium, particularly under the action of bile salts and pancreatin, which could reflect the lowest value of the bioaccessible compounds observed in the case of flavonols[Citation40] that may be the case also in the present work. For Gonzales et al.,[Citation39] the loss of certain types of flavonoid glycosides during intestinal digestion may be due to the binding of flavonoids to α-amylase and other digestive substances, this could lead to the lowest bioaccessibility of flavonoids. The bioaccessible tannins varied significantly (p < .05) among varieties during the harvest, with the values varied between 80.27 and 2242.26 μg/g for the IRAD4115 variety at 15 MAP and the EN variety at 6 MAP, respectively (). Similarly, bioaccessible resveratrol of leaves varied among the varieties with the highest value of 432.68 μg/g for the EN variety at 6 MAP and the lowest value of 1.56 μg/g for the AD variety at 15 MAP. The highest (3848.19 μg/g) and lowest (12.28 μg/g) levels of bioaccessible diosgenin were shown by the EN variety at 6MAP and 12MAP, respectively (). Leaves harvested at 6MAP showed the highest levels in the majority of cases, suggesting that the matrices are favorable to the action of digestive enzymes. It has been reported by Alminger et al.[Citation32] that the bioaccessibility of polyphenols is influenced by their particle size, structure, pH and dietary fiber. Thus, the results suggest that the leaves of the EN harvested at 6MAP could have the best structure, particle size for the release and solubilization of most polyphenols.
Table 4. Individual bioaccessible stilbenes, saponins and tannins content (simulated digested sample). Value expressed in microgram per gram dry weight
Antidiabetic and anti-obesity inhibitory activities
α-glucosidase inhibitory activity of bound, free and bioaccessible polyphenols
The α-glucosidase inhibitory effect of bound and free phenolics varied significantly (p < .05) during harvesting time (). The highest α-glucosidase inhibitory effect of bound fraction was observed in TMS92/0326 variety (1.65 mg ACE/g) at 6 MAP. This effect against α-glucosidase may be attributed to high concentration of TFC and TTC (). In fact, the TFC has been reported as the most active polyphenols against type 2 diabetes.[Citation6,Citation7] The free polyphenolic of the IRAD4115 variety showed the highest activity (1.16 mgACE/g) against α-glucosidase at 9MAP (). The α-glucosidase inhibitory effect of bound and free phenolics was significantly high at 6 and 9 MAP, respectively, which coincided with dry season. It was noted that the inhibitory effect of free polyphenols against α-glucosidase was better than bound phenolics, this result may be due to the lowest concentration of bound fraction and good structural configuration of phenolics in the free fraction extract (). Furthermore, the various types of polyphenols in the free extracts may exert their synergism to inhibit more enzyme. It is reported that phenolics content have synergistic effect on key enzymes of digestion.[Citation4,Citation41] However, the lowest activity of bound and free polyphenols against α-glucosidase was observed with the EN variety at 6MAP ().
Table 5. α-glucosidase, α-amylase and lipase inhibitory activity of bound and free polyphenols (undigested sample)
The α-glucosidase inhibitory activity of bioaccessible polyphenols varied considerably during leaf harvests (). Bioaccessible polyphenols of the TMS92/0326 variety harvested at 6MAP are more active (2.51 mgACE/g), followed by that of leaves of TMS92/0326 (3.32 mgACE/g). The concentration of tannins, flavonoids, saponins and stilbenes may justify this important inhibitory activity with regard to α-glucosidase inhibitory activity. Quercetin was known as a α-glucosidase inhibitory activity like Acarbose; thus, it can delay glucose absorption and prevent the digestion of carbohydrates by inhibiting the sucrase, maltase and α-amylase.[Citation42,Citation43] It also promotes proliferation of the pancreatic beta cell[Citation42] thus improve absorption of glucose.
Figure 1. Effect of bioaccessible (simulated digested sample) polyphenols on key enzymes: α-amylase (a), α-glucosidase (b) and lipase (c).
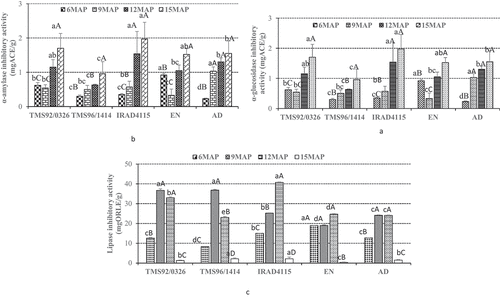
These results were similar to those obtained by Kwon et al.[Citation44] who found the inhibitory activities of these above mentioned compounds on α-glucosidase. Whereas the extracts of leaves harvested at 15 MAP of TMS92/0326 variety exhibited the weakest inhibitory effect. This suggests that the structural conformation of phenolics against α-glucosidase activity at this harvesting could not be favorable for the active site of the enzyme.
α- amylase inhibitory activity of bound, free and bioaccessible polyphenols
The α- amylase inhibitory activity of bound and free phenolics varied significantly (p < .05) throughout the harvests (). The bound polyphenols of TMS96/1414 variety (1.03 mg ACE/gdw) were more active against α-amylase activity at 15 MAP compared to other varieties, although it was statistically similar to those EN variety (1.07 mgACE/g) at 12 MAP. The free polyphenols of EN variety (0.73 mgACE/g) were more effective against α- amylase activity at 15 MAP as compared to other harvests. These results were correlated with the content of flavonoids and tannins during harvesting (). The results were also in accordance with those reported by Liu et al.[Citation6] and Rienks et al.[Citation7] who found that TFCs were more active in type 2 diabetes than the others polyphenols. Similarly, the results were consistent with those of Tan et al.[Citation27] Furthermore, flavonoids were reported as key contributors for α-amylase inhibitory activity.[Citation45] The bound and free polyphenols of TMS92/0326 (3.21 mgACE/g) and TMS96/1414 varieties (38.85 mgACE/g) observed at 9 MAP and 6 MAP, respectively, showed the lowest activity against α-amylase.
The α-amylase inhibitory activity of bioaccessible polyphenols of leaves harvested at 6MAP of AD variety was significantly (p < .05) active (0.23 mgACE/g) followed by that of TMS92/0326 variety (0.30 mgACE/g) (). However, these disparities may be linked with the solubilization of polyphenols in the best structural configuration of the enzyme site. The α-amylase inhibitory activity was low with the bioaccessible polyphenols in leaves of the IRAD4115 variety (1.97 mgACE) at 15 MAP. This result suggests that the structural configuration of bioaccessible phenolics is not in favor for α-amylase inhibitory activity. The impact of the structure of phenolics on the action of enzymes of digestion was reported.[Citation46] The strong inhibitory activity of α-amylase may link to the flavonoid contents of the extracts. In fact, it has been reported by Oboh et al.[Citation45] that the inhibitory effect of flavonoids on α-amylase activity. Flavonoids such as quercetin and kaempferol protect and increase the regeneration and viability of destructed pancreatic β-cells[Citation47] thus improve the insulin secretion.
Lipase inhibitory activity of bound, free and bioaccessible polyphenols
The lipase inhibitory activity of bound and free polyphenols also varied significantly (p < .05) during the harvests. However, the bound phenolics of TMS92/0326 variety (0.71 mgORLE/g) showed the best inhibitory activity against lipase, which was followed by TMS96/1414 (0.74 mgORLE/g) and IRAD4115 (0.75 mgORLE/g) at 6MAP (). These results were consistent with those that reported that flavonoids were responsible for the lipase inhibition.[Citation41,Citation48] The free polyphenols of TMS96/1414 variety (4.57 mgORLE/g) were more potent against lipase activity at 15 MAP. The bound and free polyphenols of TMA96/1414 (8.40 mgORLE/g) and IRAD4115 varieties (6.49 mgORLE/g) were found to be least active against lipase activity at 9MAP and 6MAP, respectively. Similarly, it was reported that the efficiency of polyphenols depends on the structures of polyphenols.[Citation42] However, it was demonstrated that the plasma total cholesterol, triglycerides, and low-density lipoprotein-cholesterol may be reduced by flavonoids and other phenolics.[Citation49] The results revealed that the harvest had a significant influence on phenolics; hence, their lipase inhibitory activity was also significantly affected.
The bioaccessible polyphenols of leaves harvested at 15 MAP of the EN variety were significantly (p < .05) active (0.39 mg ORLE/g) followed by those of the TMS92/0326 variety (1.33 mg ORLE/g) (). This strong inhibitory activity observed at 15 MAP may suggest that the bioaccessible polyphenols of the leaves at this stage of maturity could be more favorable for blocking the active sites of the lipase. The presence of certain compounds could be formed during gastrointestinal digestion that had strong lipase inhibitory activity than the methanolic extract. These results were reported by Liang et al.[Citation50] who found that cyanidin-3-glycoside and cyanidin-3-rutinoside produced 18 phenolic compounds after gastrointestinal digestion. The low inhibitory activity of the bioaccessible polyphenols shown by leaves of the IRAD4115 variety (40.71 mg ORL/g) was weak. These variations are linked to the complexity of the sample matrix, which is difficult to access for digestive enzymes to release bioactive polyphenols. Interestingly, the bioaccessible polyphenols showed the highest inhibitory activity of α-glucosidase and lipase comparable to bound and free phenolics, while the α-amylase would be the least affected.
Conclusion
This study revealed a significant (p < .05) variation of phenolics content in bound, free and bioaccessible fractions and their inhibitory potential in key enzymes of digestion. The results showed that bound fraction of phenolics was significantly low in TFC and TTC than free fraction. The bound phenolics showed a promising inhibition activity against α-amylase throughout the harvest, and also bound phenolics was more potent against lipase activity than the free fraction of phenolics. The bioaccessible phenolics compounds are lower than bound and free fractions; however, there were highly active against α-amylase and lipase compared to bound and free fractions of phenolics. Edible cassava leaves harvested at 6 MAP can be used in the management of obesity and the advance maturity stage leaves in the prevention of type 2 diabetes. Furthermore, these results suggest that cassava leaves can be used for therapeutic diets in the prevention of type 2 diabetes and obesity. Thus, edible cassava leaves have important biological active compounds, which can be used in the prevention and the management of metabolic diseases and hence can be used for pharmaceutical purposes. Our future work will focus on in vitro study of inhibitory activities of isolated bound flavonoids and stilbenes.
Abbreviations
ACE: Acarbose equivalent
DNS: 3,5-Dinitrosalicylic acid
HPLC-DAD: High-Performance Liquid Chromatography with Diode-Array Detection
IITA: International Institute of Tropical Agriculture
IRAD: Institute of Agricultural Research for Development
MAP: months after planting
ORLE: orlistat equivalent
pNPB: p-Nitrophenyl butyrate
p-NPP: p-Nitrophenol palmitate
TFC: Flavonoid contents
TTC: Total tannin contents
Data availability
Data are available from the corresponding author on reasonable request.
Author contributions
Alphonse Laya designed, performed experiments, analyzed data, and wrote the article. Benoît B. Koubala and Singh Negi Pradeep designed the research study and both contributed to the critical revision of the article.
Supplemental Material
Download MS Word (980.3 KB)Acknowledgments
The LA acknowledges CSIR-TWAS for award of fellowship to undertake this work. The author also thanks CSIR-Central Food Technological Research Institute, Mysore (India), for the instrument facilities.
Supplemental data
Supplemental data for this article can be accessed on the publisher’s website
Disclosure statement
No potential conflict of interest was reported by the author(s).
Additional information
Funding
References
- Grochowski, D. M.; Uysal, S.; Aktumsek, A.; Granica, S.; Zengin, G.; Ceylan, R.; Locatelli, M.; Tomczyk, M. In Vitro Enzyme Inhibitory Properties, Antioxidant Activities, and Phytochemical Profile of Otentilla Thuringiaca. Phytochem. Lett. 2017. DOI: 10.1016/j.phytol.2017.03.005.
- WHO, World Health Organization. Global Report on Diabetes. http://apps.who.int/iris/bitstream/10665/204871/1/9789241565257_eng.pdf,2016 ( accessed 2020).
- Burton-Freeman, B. M.; Sandhu, A. K.; Edirisinghe, I. Red Raspberries and Their Bioactive Polyphenols: Cardiometabolic and Neuronal Health Links 1,2. Am. Soc. Nutri. Adv. Nutr. 2016, 7, 44–65.
- Ozer, B.; Kirkan, M. S.; Sarikurkcu, C.; Cengiz, M.; Ceylan, O.; Atılgan, N.; Tepe, B. Onosma Heterophyllum: Phenolic Composition, Enzyme Inhibitory and Antioxidant Activities. Ind. Crops. Produ. 2018, 111, 179–184. DOI: 10.1016/j.indcrop.2017.10.026.
- Shamloo, M.; Peter, J. H.; Eck, P. Inhibition of Intestinal Cellular Glucose Uptake by Phenolics Extracted from Whole Wheat Grown at Different Locations. J. Nutri. Metabol. 2018. Article ID 5421714. doi:10.1155/2018/5421714.
- Liu, Q.; Hu, H. J.; Li, P. F.; Yang, Y.-B.; Wu, L.-H.; Chou, G.-X.; Wang, Z.-T. Diterpenoids and Phenylethanoid Glycosides from the Roots of Clerodendrum Bungei and Their Inhibitory Effects against Angiotensin Converting Enzyme and α-glucosidase. Phytochem. 2014, 103, 196–202. DOI: 10.1016/j.phytochem.2014.02.015.
- Rienks, J.; Barbaresko, J.; Oluwagbemigun, K., Schmid, M.; Nöthlings, U. Polyphenol Exposure and Risk of Type 2 Diabetes: Dose-response Meta-analyses and Systematic Review of Prospective Cohort Studies. Am. J. Clin. Nutri. 2018, 108, 1–13.
- Kim, B.; Woo, S.; Kim, M.-J.; Kwon, S.-W.; Lee, J.; Sung, S. H.; Koh, H.-J. Identification and Quantification of Flavonoids in Yellow Grain Mutant of Rice (Oyza Sativa L). Food Chem. 2018, 241, 154–162. DOI: 10.1016/j.foodchem.2017.08.089.
- Yang, M.-L.; Lu, C.; Fan, Z.-F.; Zhao, T.-R.; Cheng, -G.-G.; Wang, Y.-D.; Cao, J.-X.; Liu, Y.-P. Hypoglycemic and Hypolipidemic Effects of Epigynum Auritum in High Fat Diet and Streptozotocin-induced Diabetic Rats. J. Ethnopharmacol. 2022, 288, 114986. DOI: 10.1016/j.jep.2022.114986.
- Fernández-Quintela, A.; Milton-Laskibar, I., Aguirre, L. Effects of Quercetin on Mitochondriogenesis in Skeletal Muscle: Consequences for Physical Endurance and Glycemic Control. Int. Nutr. Skel. Musc. 2019, 505–516.
- Balamurugan, T.; Anbuselvi, S. Physicochemical Characteristics of Manihot Esculenta Plant and Its Waste. J. Chem. Pharma Res. 2013, 5(2), 258–260.
- Saad, R.; Appalasamy, L.; Khan, J.; Kazi, H.; Yusuf, E.; Asmani, F. Phytochemical Screening and Antibacterial Activity of Five Malaysian Medicinal Plants. Brit. J. Pharma Res. 2014, 4(17), 2019–2032.
- Koubala, B. B.; Laya, A., Massai, H.; Kouninki H.; Nukenine, E.N. Physico-chemical Characterization Leaves from Five Genotypes of Cassava (Manihot Esculenta Crantz) Consumed in the Far North Region (Cameroon). Am J. Food Sci. Technol. 2015, 3(2), 40–47.
- Oresegun, A.; Fagbenro, O. A.; Ilona, P.; Bernard, E. Nutritional and Anti-nutritional Composition of Cassava Leaf Protein Concentrate from Six Cassava Varieties for Use in Aqua Feed. Cog. Food. Agri. 2016, 2, 1147323.
- Chandrika, U. G.; Svenberg, U.; Jansz, E. R. Content and in Vitro Accessibility of β-carotene from Cooked Sri Lankan Green Vegetables and Their Estimated Contribution to Vitamin A Requirement. J. Sci. Food. Agri. 2006, 86, 54–61. DOI: 10.1002/jsfa.2307.
- Chandrika, R.; Sarawasthi, K. J. T.; Shivakameshwari, M. N. Phonological Events of Eryngium Foetidum L. From Karnataka, India. Int. J. Plant Reprod. Biol. 2013, 5(1), 89–91.
- Shahidi, F.; Yeo, J. D. Insoluble-bound Phenolics in Food. Molecules. 2016, 21, 1216–1237. DOI: 10.3390/molecules21091216.
- Laddomada, B.; Durante, M.; Minervini,; Minervini, F.; Garbetta, A.; Cardinali, A.; D’Antuono, I.; Caretto, S.; Blanco, A.; Mita, G., et al. Phytochemical Characterization and Anti-inflammatory Activity of Extracts from the Whole-meal Flour of Italian Durum Wheat Cultivars. Int. J. Mol Sci. 2015, 16, 3512–3527. DOI: 10.3390/ijms16023512.
- Giordano, D. B. T.; Gagliardi, F.; Blandino, M. Influence of Agricultural Management on Phytochemicals of Colored Corn Genotypes (Zea Mays L.)-Part II: Sowing Time. J. Agri. Food Chem. 2018. DOI: 10.1021/acs.jafc.8b00326.
- IITA. Pre-emptive Management of the Virulent Cassava Mosaic Disease in Nigeria. Annual report. Ibadan, Nigeria, 2005.
- Chen, G.-L.; Zhang, X.; Chen, S.-G.; Han, M.-D.; Gao, Y.-Q. Antioxidant Activities and Contents of Free, Esterified and Insoluble-bound Phenolics in 14 Subtropical Fruit Leaves Collected from the South of China. J. Fun Foods. 2017, 30, 290–302. DOI: 10.1016/j.jff.2017.01.011.
- Laya, A., and Koubala, B. B. Polyphenols in Cassava Leaves (Manihot Esculenta Crantz) and Their Stability in Antioxidant Potential after in Vitro Gastrointestinal Digestion. Heliyon. 2020, 6(3), e03567. DOI: 10.1016/j.heliyon.2020.e03567.
- Ydjedd, S.; Bouriche, S.; López-Nicolás, R.; Sánchez-Moya, T.; Frontela-Saseta, C.; Ros-Berruezo, G.; Rezgui, F.; Hayette Louaileche, H.; Kati, D-E. Effect of in Vitro Gastrointestinal Digestion on Encapsulated and Nonencapsulated Phenolic Compounds of Carob (Ceratonia Silique L.) Pulp Extracts and Their Antioxidant Capacity. J. Agric. Food Chem. 2017, 1–9. DOI:10.1021/acs.jafc.6b05545.
- Xiong, L.; Yang, J.; Jiang, Y.; Lu, B.; Hu, Y.; Zhou, F.; Mao, S.; Shen, C. Phenolic Compounds and Antioxidant Capacities of 10 Common Edible Flowers from China. J. Food Sci. 2011, 79(4), C517–C525.
- Gaytan-Martínez, M.; Cabrera-Ramírez, A. H.; Morales-Sanchez, E.; Ramírez-Jiménez, A. K.; Cruz-Ramírez, J.; Campos-Vega, R.; Velazquez, G.; Loarca-Piña, G.; Mendoza, S. Effect of Nixtamalization Process on the Content and Composition of Phenolic Compounds and Antioxidant Activity of Two Sorghums Varieties. J. Cer. Sci. 2017, 77, 1–8. DOI: 10.1016/j.jcs.2017.06.014.
- Zhang, L.; Hogan, S.; Li, J.; Sun, S.; Canning, C.; Zheng, S. J.; Zhou, K. Grape Skin Extract Inhibits Mammalian Intestinal A-glucosidase Activity and Suppresses Postprandial Glycemic Response in Streptozocin-treated Mice. Food Chem. 2011, 126, 466–471. DOI: 10.1016/j.foodchem.2010.11.016.
- Tan, Y.; Chang, S. K. C.; Zhang, Y. Comparison of α-amylase, α-glucosidase and Lipase Inhibitory Activity of the Phenolic Substances in Two Black Legumes of Different Genera. Food Chem. 2017, 214, 259–268. DOI: 10.1016/j.foodchem.2016.06.100.
- Yang, X.-J.; Dang, B.; Fan, M.-T. Free and Bound Phenolic Compound Content and Antioxidant Activity of Different Cultivated Blue Highland Barley Varieties from the Qinghai-Tibet Plateau. Molecules. 2018, 23, 872–879. Syst. Ecol. 2003;31, 3–16.
- Bhandari, S. R.; Kwak, J.-H. Seasonal Variation in Phytochemicals and Antioxidant in Different Tissues of Various Broccoli Cultivars. Afr. J. Biotechnol. 2014, 13(4), 604–615. DOI: 10.5897/AJB2013.13432.
- Williamson, G.; Clifford, M. N. Clifford, Role of the Small Intestine, Colon and Microbiota in Determining the Metabolic Fate of Polyphenols. Biochem. Pharma. 2017, 34, 65–73.
- Ossipov, V.; Salminen, J.-P., Ossipova, S.; Haukioja, E.; Pihlaja, K. Gallic Acid and Hydrolysable Tannins are Formed in Birch Leaves from an Intermediate Compound of the Shikimate Pathway. Biochem Syst. Ecol, 2003, 31, 3–16.
- Alminger, M.; Aura, A-M.; Bohn, T.; Dufour, S N E.; Gomes, A.; Karakaya, S.; Martínez-Cuesta, M C.; McDougall, G J.; Requena, T.; Santos, CN. In Vitro Models for Studying Secondary Plant Metabolite Digestion and Bioaccessibility.13. Compreh Rev.Food Sci. Food Saf. 2014, 43, 23–30.
- Gouvea, D. R.; Gobbo-Neto, L.; Sakamoto,; Sakamoto, H. T.; Lopes, N. P.; Lopes, J. L. C.; Meloni, F.; Amaral, J. G. Seasonal Variation of the Major Secondary Metabolites Present in the Extract of Erementhus Mattogrossensis Less (Astreraceae: Vernonieae) Leaves. Quim. Nova. 2012, 35, 2139–2145. DOI: 10.1590/S0100-40422012001100007.
- Ernst, W. H.; Kuiters, A. T.; Nelissen,; Nelissen, H. J. M.; Tolsma, D. J. Seasonal Variation in Phenolics in Several Tree Species in Botswana. Acta Bot. Neerl. 1991, 40, 63–74. DOI: 10.1111/j.1438-8677.1991.tb01514.x.
- Anttonen, M. J.; Hoppula, K. I.; Nestby, R.; Verheul, M. J.; Karjalainen, R. O. Influence of Fertilization, Mulch Color, Early Forcing, Fruit Order, Planting Date, Shading, Growing Environment, and Genotype on the Contents of Selected Phenolics in Strawberry (Fragaria×ananassafragaria × Ananassa Duch.) Fruits. J. Agric. Food Chem. 2006, 54, 2614–2620. DOI: 10.1021/jf052947w.
- Jaakola, L.; Määttä-Riihinen, K.; Kärenlampi, S.; Hohtola, A. Activation of Flavonoid Biosynthesis by Solar Radiation in Bilberry (Vaccinium Myrtillus L.) Leaves. Planta. 2004, 218, 721–728. DOI: 10.1007/s00425-003-1161-x.
- Bujor, O. C.; Giniès, C.; Popa,; Popa, V. I.; Dufour, C. Phenolic Compounds and Antioxidant Activity of Lingonberry (Vaccinium Vitis-idaea L.) Leaf, Stem and Fruit at Different Harvest Periods. Food Chem. 2018. DOI: 10.1016/j.foodchem.2018.01.052.
- Khanam, U. K. S.; Oba, S.; Yanase, E.; Murakami, Y. Phenolic Acids, Flavonoids and Total Antioxidant Capacity of Selected Leafy Vegetables. J. Funct Foods. 2012, 4(4), 979–987.
- Gonzales, G. B.; Smagghe, G.; Grootaert, C.; Zotti, M.; Raes, K.; Camp, J. V. Flavonoid Interactions during Digestion, Absorption, Distribution and Metabolism: A Sequential Structure–activity/property Relationship-based Approach in the Study of Bioavailability and Bioactivity. Drug Metabol. Rev. 2015, 47(2), 175–190.
- Bouayed, J.; Hoffmann, L.; Torsten, B. Total Phenolics, Flavonoids, Anthocyanins and Antioxidant Activity following Simulated Gastro-intestinal Digestion and Dialysis of Apple Varieties: Bioaccessibility and Potential Uptake. Food Chem. 2012, 128, 14–21. DOI: 10.1016/j.foodchem.2011.02.052.
- McDougall, G. J.; Kulkarni, N. N.; Stewart, D. Current Developments on the Inhibitory Effects of Berry Polyphenols on Digestive Enzymes. Bio Fact. 2008, 34(1), 73–80.
- Adewole, S. O.; Caxton-Martins, E. A.; Ojewole, J. A. Protective Effect of Quercetin on the Morphology of Pancreatic β-cells of Streptozotocin-treated Diabetic Rats. Afr. J. Tradit. Complement. Altern. Med. 2007, 4(1), 64–74. DOI: 10.4314/ajtcam.v4i1.31196.
- Soltesova-Prnova, M.; Milackova, I.; Stefek, M. 3′-O-(3-Chloropivaloyl) Quercetin, α-glucosidase Inhibitor with Multi-targeted Therapeutic Potential in Relation to Diabetic Complications. Chem. Pap. 2016, 70(11), 1439–1444. DOI: 10.1515/chempap-2016-0078.
- Kwon, Y.-I.; Apostolidis, E.; Shetty, K. Evaluation of Pepper (Capsicum Annuum) for Management of Diabetes and Hypertension. J. Food Biochem. 2007, 31(3), 370–385. DOI: 10.1111/j.1745-4514.2007.00120.x.
- Oboh, G.; Agunloye, O. M.; Adefegha, S. A., Akinyemi A. J., Ademiluyi A. O. Caffeic and Chlorogenic Acids Inhibit Key Enzymes Linked to Type 2 Diabetes (In Vitro): A Comparative Study. J. Basic Clin. Physiol. Pharma. 2015, 26(2), 165–170.
- Rosicka-Kaczmarek, J. Polifenole jako naturalne antyoksydanty w_ zywnos´ci,”. Przeg Piek. Cuk. 2004, 6, 12–16.
- Eldaim, M. A. A.; Elrasoul, A. S. A.; Elaziz, S. A. A. Moringa Oleifera Leaves Aqueous Extract Ameliorates Hepatotoxicity in Alloxan- Induced Diabetic Rats. Biochem. Cell Biol. 2017, 95(4), 524–530. DOI: 10.1139/bcb-2016-0256.
- De Pradhan, I.; Dutta, M.; Choudhur, K.; De, B. Metabolic Diversity and in Vitro Pancreatic Lipase Inhibition Activity of Some Varieties of Mangifera Indica L. Fruits. Int. J. Food Prop. 20 2017, S3, S3212–S3223. DOI: 10.1080/10942912.2017.1357041.
- Amina, M. D.; Attakpa, S. E.; Machioud, S. M.; Félix, G.; Rodrigue, A.; Abdou Madjid, A.; Latifou, L.; Lamine, B.-M.; Seri, B.; Akhtar Khan, N., et al. Molecular Mechanisms of Hypoglycemic and Antioxidative Effects of Phyllanthus Amarus on Streptozotocin-Induced Diabetic Rats. J. Endocrinol. Diab. 2018, 5(4), 1–16. DOI: 10.15226/2374-6890/5/4/01112.
- Liang, L.; Wu, X.; Zhao, T.; Zhao, J.; Li, F.; Zou, Y.; Mao, G.; Yang, L. In Vitro Bioaccessibility and Antioxidant Activity of Anthocyanins from Mulberry (Morus Atropurpurea Roxb.) Following Simulated Gastrointestinal Digestion. Food Res. Inter. 2012, 46, 76–82. DOI: 10.1016/j.foodres.2011.11.024.