ABSTRACT
This study aimed to purify and identify a novel antidiabetic peptides from Chinese giant salamander (Andrias davidianus) protein hydrolysate and to evaluate its anti-diabetic properties against α-amylase and α-glucosidase. The isolation was done by gel filtration, reverse phase chromatography (RF-HLPC), and finally peptide and amino acid sequences were identified by LC/MS/MS system. Our results revealed five novel peptides that strongly inhibited α-amylase and α-glucosidase. The peptides’ amino acid sequences were Cys-Ser-Ser-Val (MW = 393.99 Da), Tyr-Ser-Phe-Arg (MW = 570.99 Da), Ser-Ala-Ala-Pro (MW = 343.89 Da), Pro-Gly-Gly-Pro (MW = 325.99 Da) and Leu-Gly-Gly-Gly-Asn (MW = 415.99 Da) possessing α-amylase inhibitory activity IC50 of 13.76 × 103, 10.82 × 103, 4.46 × 103, 4.23 × 103, and 2.86 × 103 µg/mL, respectively; and for α-glucosidase with IC50 of 206.00, 162.00, 66.90, 63.50, and 42.93 µg/mL, respectively. Intriguingly, the peptide LGGGN showed higher inhibition on both α-amylase and α-glucosidase and could be considered as a potential anti-diabetic inhibitor.
Introduction
Diabetes mellitus is one of the metabolic disorders which draw greater health concern worldwide due to its prevalent and fastest increase.[Citation1,Citation2] Typically, type 2 diabetes accounts 90–95% cases are anticipated to reach 221 million[Citation3] or 366 million by 2030[Citation2]. Several reasons contribute to the persist and quick raising of this chronic disease owing to the genetic or acquired insulin secretion deficiency marked by diminishing of the pancreatic islet responsiveness in insulin production. This incompleteness results in a high level of plasma glucose and consequently lead to the distortion of the immune system, blood vessel, and nerve.[Citation4,Citation5] Moreover, lifestyle change associated with bad eating habits, less exercise, and aging is another hallmark for the ever-increasing of diabetic especially type 2.[Citation3] Therefore, paying more attention in diagnosis and assure suitable treatments is required to prevent or reduce diabetes-related short-term consequences and complications such as eye sight problem, tiredness, and hyperglycemia and long-term ones like chronic renal failure, cardiovascular disease, and neuropathy.[Citation6]
Figure 1. Changes in degrees of hydrolysis (DH) and inhibition activity over hydrolysis time produced from different protease enzymes in different enzyme concentration. A 0.04 E/S, B 0.08 E/S, C 0.1 E/S, and D Trypsin 0.1 E/S degree of hydrolysis with alpha amylase inhibition activity.
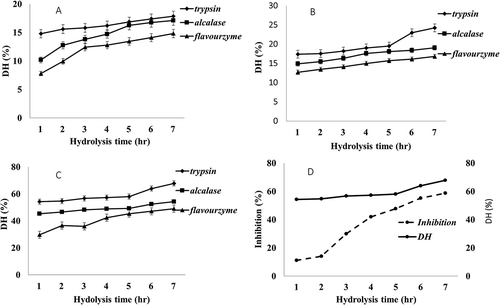
Figure 2. Separation of anti-diabetic peptides from Andrias davidianus protein hydrolysate ultrafiltration fraction by Sephadex G 25 gel filtration load in column 1.6 × 100 cm column. Sample was eluted with water at flow rate of 1 mL/min in the 220 nm wavelength region. Three peptide fractions were obtained according to molecular size distribution, fraction I, II, and III were eluted.
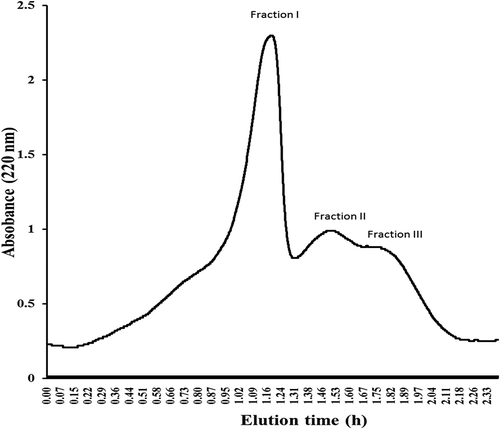
Figure 3. Chromatograms fractions obtain by HPLC with column TSKgel 2000 SWXL 300 mm×7.8mm elute by acetonitrile/water/trichloroacetic acid (TCA), 45/55/0.1 (V/V) show three pattern from fraction I, II and III in with fraction II were corresponding to the target peptide with retaining time 17.557 and 19.656 min.
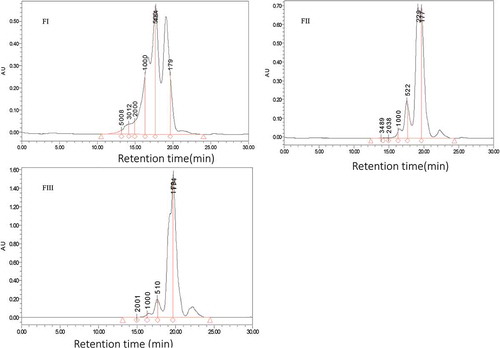
Different ways have been recommended to treat diabetes ranging from natural and synthetic way by taking chemical drug such as acarbose and metformin, change of life style by proper intake of diet and emphasize retinue exercise to control blood pressure and body over weight.[Citation6,Citation7] All these factors are linked in controlling blood sugar which is an essential part of managing diabetes; however head-to-head comparison of diabetes medications showed severe drawbacks.[Citation8] To a considerable degree, researchers have focused on searching for anti-diabetic compounds from natural materials which could act as an anticatalyst to compete with α-amylase and α-glucosidase that can prevent hyperglycemia without any side effects.[Citation9] Consistently, repression of these enzymes has been medically adopted to slow down glucose absorption resulting in inhibition of postprandial hyperglycemia. Nevertheless, restraint in carbohydrate digestion by the application of natural inhibitors is still considered as an alternative treatment for diabetes.[Citation6]
Food derived bioactive peptides as natural materials are adequate for inhibiting α-amylase and α-glucosidase satisfactorily with more other additional effects including antihypertensive, antioxidant, anti-microbial and anti-proliferative.[Citation10–Citation16] Enzyme hydrolysis is the promising technique in the production of bioactive peptide with higher activities compared to its precursors. Many studies underlying the beneficial and mechanism of the bioactive peptide in type 2 diabetes prevention have been elucidated.[Citation5,Citation17] For instance, anti-diabetic peptide from animal source comprises Sardine,[Citation18,Citation19] Shark liver,[Citation20] Atlantic salmon skin gelatin,[Citation21] Silver Carp,[Citation2] Cocoon and Silkworm,[Citation22,Citation23] Egg white, and Yolk.[Citation6,Citation24–Citation26] Notably, a numbers of studies have suggested plant like Guava leaves,[Citation27] Selaginella tamariscina,[Citation28] Phaseolus coccineus,[Citation29] and Nepalese medicinal Bergenia ligulata[Citation4] to have anti-diabetic properties. Based on previous research findings there is no report on anti-diabetic compounds from Chinese giant salamanders (Andrias davidianus). Therefore, the present study report the isolation and identification of the bioactive peptides from Andrias davidianus muscle obtained through enzymatic hydrolysis. The α-amylase and α-glucosidase inhibitory activity is evaluated by anti-diabetic test, followed by prediction of chemical sequences of bioactive peptides which could be used in the management of type 2 diabetes.
Materials and methods
Materials
2.4 L Alcalase from Bacillus licheniformis with activity of 2.4 AU/g and Trypsin (EC 3.4.21.4) from porcine pancreas were purchased from Sinopharm chemical reagent Co., Ltd and Flavorzyme from Aspergillus oryzae was purchased from Sigma-Aldrich Co., Ltd. All these enzymes were used to prepare the protein hydrolyses with deference conditions. Male healthy Chinese giant salamanders (Andrias davidianus) with three or four years old, body length of 70–100 cm and 4 kg of weight was provided by Yangzhou Rongda Agricultural Science and Technology Co., Ltd (Jiangsu, China). All chemicals used were analytical reagent grade.
Determination of chemical composition
Chemical composition (protein content, fat ash and moisture) was examined according to the method of (AOAC 1990). Protein content was determined by Kjeldahl method using 6.25 nitrogen protein conversion factor as described by the method of[Citation30] with slight modifications. The total lipid content was determined using the Soxhlet extraction method with petroleum ether. Moisture content was examined by evaporation method under 150ºC until an unchanged weight recorded. The total ash content was examined by heating sample to constant mass with muffle furnace at a temperature of 550°C. All analysis was performed in triplicate.
Preparation of protein hydrolysates (PH)
Protein hydrolysates was prepared by three different selected enzymes under temperature and pH conditions () in connected jacketed reaction vessel of 250 mL equipped with stirrer (IKA C-MAG HS 4 S25) and a super constant temperature stirring thermostatic water baths (Shanghai Blue pard Yiheng Technical Co., Ltd). Before addition of enzyme, slurry was homogenized and adjusted to the desired pH and temperature which were kept constant for all enzyme hydrolysis followed by the reaction of 420 min. Subsequently, the aliquot of 10 mL were drawn every 60 min from the reaction mixture to examine the degree of hydrolysis. To inactivate the enzyme, the solution was heated, centrifuged at a speed of 10,000 rpm at 4 ºC for 15 min, and finally supernatants were dialyzed and freeze dried to get pulverized hydrolysates prior for incubation at −20 until further analysis.
Table 1. Hydrolysis conditions for preparation of protein hydrolysate of Andrias davidianus.
Determination of degree of hydrolysis
Degree of hydrolysis is described as percentage of free amino group cleaved from protein, which is measured from the ratio of α-amino nitrogen (AN) and the total nitrogen (TN).[Citation30] The AN was examined using the method of formal titration as reported previously[Citation31] with slight modifications, equal amount of sample solution (PH) and water 10 mL (w/w) was mixed and adjusted to pH 7.5 using 0.1 M NaOH followed by 10 mL of formaldehyde 38% (v/v) and left for 5 min in room temperature. Titration was conducted to the end point at the pH 9.0 using standard NaOH solution and the volume consumed was used to calculate the amount of free amino group. DH was calculated by dividing amount of free amino acid group and the total amount of nitrogen. All Analysis were performed in triplicate.
Separation of peptide by column chromatography
Powdered hydrolysates was fractioned by gel chromatography equipped with detector (DH-3000) and pump (DH-A) Shanghai Jiapeng Technology Co., Ltd with Sephadex G 25 gel (GE healthcare Life science) load in column 1.6 × 100cm. Pre-equilibration was done by double water and calibrated with lyophilized powder Bovine Serum Albumin as a gel filtration molecular weight standard with molecular weight of 66 kDa (Sinopharm Chemical Reagent Co., Ltd). Then, the hydrolysates of 10 mg/mL was eluted at 1.0mL/min and 220 nm absorbance. Fragments were collected according to molecular weight, lyophilized and kept under −20 °C prior for further study. Meanwhile, all the fractions were analyzed for anti-diabetic activity.
Determination of peptide molecular weight distribution
The fractions obtained from gel filtration chromatography were subjected to high performance liquid chromatography (HPLC) to determine their molecular weight distribution on each fraction. This parameter indicates how well hydrolysis of protein occurred and its association with bioactive peptide fractions (Chen, Chi et al. 2011). Analysis was carried out by HPLC (Waters 600) with column TSKgel 2000 SWXL 300mm×7.8mm and mobile phase acetonitrile/water/trichloroacetic acid (TCA) of 45/55/0.1(V/V), respectively. Before injection of the sample, the fractionated powder was dissolved in mobile phase buffer, centrifuged for 15 min at 10,000 rpm and then filtered with 0.45 µm membrane. Subsequently, the sample was eluted at 0.5mL/min flow rate and monitored with 220 nm UV monitor and column temperature of 30 °C. As standards, Cytochrome C MW 12384 Da, Bacitracin MW 1450 Da, Gly-Gly-Tyr-Arg MW 451Da, and Gly-Gly-Gly MW189 Da were used to prepare calibration curve of molecular weight peptide
Determination of amino acid composition
Higher active peptide fraction was analyzed for the amino acid composition using amino acid analyzer. Briefly, 6 N hydrochloric acid was used to hydrolyzed the sample (100 mg) for 24 h which were put in the nitrogen sealed tube and incubate in oven under 110°C. Then, the mixture was neutralized in 3.5 M NaOH, balanced with 0.02 HCl (pH 2.2) and then filtered by hydrophilic 0.45 nm membrane. Finally, 0.4 mL solutions were analyzed by amino acid analyzer (Hitachi L-8800, Tokyo, Japan). Amino acid content was expressed as the number of residues/1000 residues.
Purification of peptide by high-performance liquid chromatography (RP- HPLC)
Fraction exhibiting high anti-diabetic inhibition in gel filtration was further purified by analytical RP-HPLC with C18 column XselectTM CSH130 (4.6 × 250 cm) at the flow rate of 1 mL/min with linear gradient of 0.1% acetonitrile containing 0.0065% TFA to 20% acetonitrile containing 0.05% TFA in 35 min, 20% acetonitrile containing (0.05% TFA) to 80% acetonitrile containing (0.05% TFA) in 10 min, 80% acetonitrile (0.05 TFA) to 2 acetonitrile (0.065% TFA) in 10 min, sequentially. Eluted peptide fractions were detected at 280 nm and active fractions with different retention time were collected, lyophilized and sequenced for amino acid. Fraction with desired peaks were verified by α-amylase and α-glucosidase inhibition assay after removal of acetonitrile by evaporation.
Identification of purified peptides by LC/MS/MS
The identified peptides were analyzed by LC/MS/MS following the method of[Citation4,Citation32] with slight modifications. The LC/MS/MS is equipped with an Agilent 1200 HPLC system and 4000 Q-Trap mass spectrometer. An isocratic mobile phase was consisted of 70% acetonitrile (B) and deionized water with 0.1% trifluoroacetic acid (TFA) (A). The gradient elution program initially started from 10% B and followed by a linear gradient to 100% B in 10 min. The total mobile phase flow rate was set at 1 mL/min. Acquisition method is comprised of an information dependent data acquisition (IDA) scan cycle including an enhanced mass scan (EMS) as survey scan, an enhanced resolution scan (ER) to confirm change state and two dependent enhanced product ion (EPI) scans. With the threshold of ion intensity at 5500 counts per second (cps), the IDA criteria were set to allow the most abundant ion in the EMS scan to trigger the EPI scans. To minimize possible fragmentation in EMS, collision energy was set at 5 eV and the collision induced dissociation (CID) gas was set at low. The scan range or EMS was set at 275–1000 m/z. The dependent EPIs scan range of 275–1000 m/z; including setting for CID gas at 55 eV (GS 1) and 60 eV (GS 2) was used for the collision energy. The speed of EMS and EPI scans were recorded at the speed of 4000 Da/s.
Α-amylase inhibitory activity
The α-amylase inhibitory assay was conducted according to the method of[Citation33,Citation34] with slight modifications. Aliquots of 1.0 mL of α-amylase solution (1 unity mL−1, distilled water) was pre-mixed with 1.0 mL of sample solution at different concentrations (in 10 5 DMO). After 15 min incubation, 1% starch solution in 0.1 M phosphate buffer (pH 6.9) was added to start the reaction. This reaction was carried out at 37.5°C for 5 min and terminated by adding 600 µL dinitrosalicylic acid (DNS), a color reagent. The mixture was boiled for 15 min, cooled down at room temperature and then α-amylase activity was measured at 540 nm by spectrophotometer (Shimadzu, and Tokyo Japan). Inhibitory activity was calculated using equation 1.
where: A; optical density of reaction blank, which is the mixture containing same volume of buffer instead of sample and B; optical density of the reaction in the presence of both α-amylase and sample.
Α-glucosidase inhibitory activity
This assay was conducted according to the method of[Citation35,Citation36] with slight modifications. 7.5 µL Aliquots of the α-glucosidase solution (10 unity mL−1 0.1 M phosphate buffer pH 6.8) was pre-mixed with 15 µL of sample solution at different concentrations (in % DMSO) in 620 µL of 0.1 M phosphate buffer (pH 6.8). Then, incubation at 37.5°C for 20 min followed and 15 µL of p-nitro phenyl glucopyranoside (pNPG mM) was added as substrate to the mixture to start the reaction. The reaction mixture was incubated at 37.5°C for 30 min, followed by addition of 850 µL of 1.0 M Na2CO3 solution to terminate the reaction. The amount of released product (p-nitro phenol) was measured at 410 nm using a UV/Visible spectrometer (Ultrospec 2100 pro Amersham Bioscience UK Ltd) to estimate the enzyme activity. Inhibition activity was calculated using equation 2.
where: A; optical density of the reaction blank, the reaction mixture containing the same volume of buffer instead of sample and B; optical density of reaction in presence of both α-glucosidase and sample.
Statistical analysis
The presented data were obtained from triplicate samples and subjected to analysis of variance (ANOVA) and each value is expressed as mean ± standard deviations. Analysis of variance was carried out by SPSS program (version 19.0) at p < 0.05.
Result and discussion
Degree of hydrolysis
Values of degree of hydrolysis (DH) produced from different protease enzymes in different conditions show significance correlation with enzyme concentration and time (). The muscles’ proteins with proximate composition () were hydrolyzed for 7 h to produce comparable value of DH from 7% to 67%. The results show that there DH increases significantly with the increase of enzyme concentration and time . It is noteworthy that 0.04 enzyme/substrate ratio (E/S) shows the lowest DH compared 0.06 E/S and 0.1 E/S concentrations which produce the maximum DH value of 67.79%. Flavorzyme for example yielded the lowest 14.82% DH followed by Alcalase 17.84% DH, correlating with previous report.[Citation37] As shown in , there were a sharp increasing in DH at the first 3 h, and this increase was directly proportional to the time of hydrolysis, but after that, the rising remained nearly constant. Trypsin in 0.1 E/S accounted the higher activity offering the maximum DH of 67% . Protein hydrolysates produced by this condition were used to conduct α-amylase inhibitory activity assay in every hour time-point during hydrolysis. As shown in Figure. 1D, the highest alpha amylase inhibitory activity was 55.193% at 6 h of reaction time . For this result protein hydrolysates prepared by Trypsin 0.1 E/S was used for further study using gel filtration chromatography.
Table 2. Proximate compositions of Andrias davidianus.
Separation of peptide by size exclusion chromatography and molecular weight distribution
Three distinctive peptide peaks namely fraction I, II and III () were separated from Andrias davidianus protein hydrolysates using Sephadex G-25 gel chromatography. The molecular weight distribution of Andrias davidianus protein hydrolysates peptide fractions hydrolysed by Trypsin 0.1 E/S in 7 h was determined by HPLC showing abundance distribution of peptide molecular weight of all fractions with a molecular mass below 1000 Da (). For fraction I, II and III molecular weight peptides were distributed at 698–1318 Da (40.53%) and 316–689 Da (76.96%), 256–643 Da (65.85%) and 138–256 Da (89.78%), and 242–640 Da (52.8%) and 135–242 Da (94.26%), respectively. These results demonstrate that fraction II were more active the highest activity compared to other fractions ()
Table 3. Biological activities of the peptide fractions separated by hydrolysis, size exclusion chromatography and reverse-phase chromatography. a
Amino acid profile
The analysis of amino acids content of hydrolysate of fraction II () showed that essential and non-essential amino acid are both present in adequate amount. However, the essential amino acid were higher than the suggested by FAO/WHO for children requirement[Citation37] In the total amino acids content, hydrophilic amino acid tend to dominate the hydrophobic ones. In one hand, Glutamic acid, an aliphatic amino acid accounted higher amount with 102.4 g/kg followed by arginine (94.5 g/kg), aspartic acid (59.0 g/kg), proline (452 g/kg), glycine (33.6 g/kg), serine (29.4 g/kg), and alanine (28.4 g/kg). This findings are consistent with that obtained by (Huang and Wu Citation2010) from shark liver .On the other hand, hydrophobic amino acid content showed leucine (6.40 g/kg) dominance followed by proline (45.2 g/kg), isoleucine (43.7 g/kg), valine (40.4 g/kg), glycine (33.6 g/kg), alanine (28.4g/kg), phenylalanine (27.6 g/kg), methionine (23.2 g/kg), and tyrosine (18.1 g/kg).
Table 4. Amino acid compositions of Andrias davidianus protein hydrolysate.
The results of amino acids analysis showed that there are higher quantities of glutamic acid, leucine, isoleucine, arginine, serine, threonine, valine, and alanine suggested that protein hydrolysate of Andrias davidianus could have bioactive peptide inhibiting α-amylase activity together with α-glucosidase activity since these amino acid are associated with regulation and secretion of insulin.[Citation13,Citation38,Citation39] These amino acids, under suitable state enhance insulin secretion from primary islet cell and β cell lines.[Citation40] The study reports revealed that amino acids such as leucine and arginine may play a critical role in enhancing insulin secretion by allosteric activation of metabolism or membrane depolarization, or a combination of these possibilities.[Citation39] Various in vitro studies suggested that incubation of pancreas β-cells with Arginine, phenylalanine, leucine and Glutamic acid exhibited strong insulinotropic effects.[Citation9,Citation41] These results confirmed that specific amino acid from Andrias davidianus protein hydrolysates could have influence in the metabolic secretion of insulin from pancreatic β-cell in vivo and in vitro.
Isolation and purification of bioactive peptides
The RP HPLC purification employing C18 column resulted in 14 peptide peaks (figure not shown). These peaks were collected, concentrated, freeze dried and then examined for anti-diabetic activity. Among all fractions assayed by α-amylase and α-glucosidase only four peaks responded to inhibition and the activities were above 50%. The fractions that had activity were fraction one (F1), fraction two (F2), fraction three (F3), fraction four (F4), and fraction eight (F8). The F8 were found to have the significant highest anti-diabetic activity while F1 accounted the lowest anti-diabetic activity compared to all fractions.
Amino acid sequence and characteristics of purified peptide
Identification and amino acid sequencing of highest anti-diabetic fractions (F1, F2, F3, F4, and F8) were done by LC/MS/MS (). MS/MS spectrum of single fraction changed at the m/z with 393.99, 570.99, 343.89, 325.99, and 415.99 Da, respectively; and such results analysis revealed that the signals at m/z = 395.25 [M + H]+, 572.02 [M + H]+, 344.94 [M + H] +, 327.16 [M + H] + and 417.09 [M + H] + are responsible for the amino acid sequence of CSSV, YSFR, SAAP, PGGP, and LGGGN, respectively ().
Table 5. Amino acid sequence and characteristics of purified peptides.
Activity of peptide against α-amylase
The α-amylase inhibitory activities of the peptide sample were evaluated (). The inhibitory activity at IC50 of the fractions indicate that the F8 (2.862 × 103 µg/mL) had the highest activity compared to other fractions followed by F4 (4.234 × 103 µg/mL), F33 (4.460 × 103 µg/mL), F2 (10.824 × 103 µg/mL) and F1 (13.760 × 103 µg/mL), respectively. The peptide sequence of F8 were LGGGN composed of Leu (68%), Gly (68%), Gly (63%), Gly (63%), Asn (77%) amino acids with 81% purity. Other peptide sequence followed, whereby F4 peptide sequence was PGGP containing Pro (100%), Gly (90%), Gly (90%), Pro (99%) amino acids and its purity was 96%. The F3 peptide sequence were SAAP with Ser (68%), Ala (68%), Ala (78%), and ProM (79%) amino acids and exhibiting purity 83% of purity. The F2 peptide sequence were YSFR having Tyr (97%), Ser (83%), Phe (82%), Arg (89%) amino acids, and purity of 93%. Finally, the F1 peptide sequence were CSSV with Cys (91%), Ser (91%), Ser (89%), Val (89%) amino acids with purity of 97%. This peptide CSSV contained higher purity among all sequences while LGGGN was characterized by the least.
Property of α-glucosidase inhibitory activity
In this assay, all fractions exhibited inhibition capacity of 50%, where peptide PGGP (Figure. 3)dominated all fractions having significance IC50 value of 63.5 µg/mL which is five times lower than the original hydrolysate. The amino acid sequence of this peptide contained Gly (90%) and Pro (99%) amino acids. The presence of high Gly in this sequence could be the possible course of α-glucosidase inhibition consistent with other reports in that several amino acid can act as α-glucosidase inhibitors.[Citation23] Contrary, most of the α-glucosidase inhibitors are sugars or sugar derivatives.[Citation5] However, the most active compounds with antidiabetic potential were isolated in different materials like Try-Tyr-Pro-Leu peptide from Sardine muscle hydrolysate,[Citation42] Gln-Pro-Gly-Arg from silkworm pupae with amino acid sequence and peptide KLPGF from albumin hydrolysate.[Citation43] Nevertheless, mechanisms by which protein hydrolysates, bioactive peptides and amino acids control glucose levels is still elusive owing the involvement of complex series to archive the goal of inhibition or regulation of glucose uptake in peripheral tissue. Thus, this results provide considerable and intense demand for further study.
Conclusion
In conclusion, purification and characterization of derived peptides from Andrias davidianus protein hydrolysate conducted by enzyme hydrolysis, size exclusion chromatography and RF-HPLC leading to sequencing of five distinguished peptides namely CSSV, YSFR, SAAP, PGGP, LGGGN, and all peptides exhibited antidiabetic activity. Furthermore, we demonstrated that the peptide LGGGN had higher significance inhibitory activity on both α-amylase and α-glucosidase while the peptide PGGP particularly had the highest α-glucosidase inhibition. This study suggests that the bioactive peptides from Andrias davidianus are appropriate candidate toward investigating anti-diabetic compounds from natural food source. Thus, focus to unveil pharmacological and biochemical mechanisms of action of these peptides on diabetes management both in vivo and in vitro is of great importance.
Declaration of interest
Authors declare that they have no conflict of interest.
Additional information
Funding
References
- Gondi, M.; Basha, S. A.; Bhaskar, J. J.; Salimath, P. V.; Rao, U. J. Anti-Diabetic Effect of Dietary Mango (Mangifera Indica L.) Peel in Streptozotocin-Induced Diabetic Rats. J. Sci. Food Agr. 2015, 95, 991–999. DOI: 10.1002/jsfa.6778.
- Zhang, Y.; Chen, R.; Chen, X.; Zeng, Z.; Ma, H.; Chen, S. Dipeptidyl Peptidase IV-Inhibitory Peptides Derived from Silver Carp (Hypophthalmichthys Molitrix Val.) Proteins. J. Agric. Food Chem. 2016, 64, 831–839. DOI: 10.1021/acs.jafc.5b05429.
- Widharna, R. M.; Soemardji, A. A.; Wirasutisna, K. R.; Kardono, L. B. S. Anti Diabetes Mellitus Activity in Vivo of Ethanolic Extract and Ethyl Acetate Fraction of Euphorbia Hirta L. Herb. Int J Immunopathol Pharmacol. 2010, 6, 231–240. DOI: 10.3923/ijp.2010.231.240.
- Bhandari, M. R.; Jong-Anurakkun, N.; Hong, G.; Kawabata, J. Α-Glucosidase and Α-Amylase Inhibitory Activities of Nepalese Medicinal Herb Pakhanbhed (Bergenia Ciliata, Haw.). Food Chem. 2008, 106, 247–252. DOI: 10.1016/j.foodchem.2007.05.077.
- Makkar, F.; Chakraborty, K. Antidiabetic and Anti-Inflammatory Potential of Sulphated Polygalactans from Red Seaweeds Kappaphycus Alvarezii and Gracilaria Opuntia. Int J Food Prop. 2016, 20, 1326–1337. DOI: 10.1080/10942912.2016.1209216.
- Yu, Z. P.; Yin, Y. G.; Zhao, W. Z.; Yu, Y. D.; Liu, B. Q.; Liu, J. B.; Chen, F. Novel Peptides Derived from Egg White Protein Inhibiting Alpha-Glucosidase. Food Chem. 2011, 129, 1376–1382. DOI: 10.1016/j.foodchem.2011.05.067.
- Cui, J.; Gu, X.; Wang, F.; Ouyang, J.; Wang, J. Purification and Structural Characterization of an Alpha-Glucosidase Inhibitory Polysaccharide from Apricot (Armeniaca Sibirica L. Lam.) Pulp. Carbohydr Polym. 2015, 121, 309–314. DOI: 10.1016/j.carbpol.2014.12.065.
- Yang, W.; Liu, J.; Shan, Z.; Tian, H.; Zhou, Z.; Ji, Q.; Weng, J.; Jia, W.; Lu, J.; Liu, J.; Xu, Y.; Yang, Z.; Chen, W. Acarbose Compared with Metformin as Initial Therapy in Patients with Newly Diagnosed Type 2 Diabetes: An Open-Label, Non-Inferiority Randomised Trial. Lancet Diabetes Endocrinol. 2014, 2, 46–55. DOI: 10.1016/S2213-8587(13)70021-4.
- van Loon, L. J.; Kruijshoop, M.; Menheere, P. P.; Wagenmakers, A. J.; Saris, W. H.; Keizer, H. A. Amino Acid Ingestion Strongly Enhances Insulin Secretion in Patients with Long-Term Type 2 Diabetes. Diabetes Care. 2003, 26, 625–630. DOI: 10.2337/diacare.26.3.625.
- Ryan, J. T.; Ross, R. P.; Bolton, D.; Fitzgerald, G. F.; Stanton, C. Bioactive Peptides from Muscle Sources: Meat and Fish. Nutrients. 2011, 3, 765–791. DOI: 10.3390/nu3090765.
- Rutherfurd-Markwick, K. J.;. Food Proteins as a Source of Bioactive Peptides with Diverse Functions. Br. J. Nutr. 2012, 108(Suppl 2), S149–57. DOI: 10.1017/S000711451200253X.
- Jin, D. X.; Liu, X. L.; Zheng, X. Q.; Wang, X. J.; He, J. F. Preparation of Antioxidative Corn Protein Hydrolysates, Purification and Evaluation of Three Novel Corn Antioxidant Peptides. Food Chem. 2016, 204, 427–436. DOI: 10.1016/j.foodchem.2016.02.119.
- Singh, B.; Kaur, A. Antidiabetic Potential of a Peptide Isolated from an Endophytic Aspergillus Awamori. J Appl Microbiol. 2016, 120, 301–311. DOI: 10.1111/jam.12998.
- Nourmohammadi, E.; SadeghiMahoonak, A.; Alami, M.; Ghorbani, M. Amino Acid Composition and Antioxidative Properties of Hydrolysed Pumpkin (Cucurbita Pepo L.) Oil Cake Protein. Int J Food Prop. 2017, 1–12. DOI: 10.1080/10942912.2017.1283516.
- Vieira, E. F.; Ferreira, I. M. P. L. V. O. Antioxidant and Antihypertensive Hydrolysates Obtained from By-Products of Cannery Sardine and Brewing Industries. International. J Food Prop. 2016, 20, 662–673. DOI: 10.1080/10942912.2016.1176036.
- Huang, Y.-L.; Ma, M.-F.; Chow, C.-J.; Tsai, Y.-H. Angiotensin I-Converting Enzyme Inhibitory and Hypocholesterolemic Activities: Effects of Protein Hydrolysates Prepared from Achatina Fulica Snail Foot Muscle. Int J Food Prop. 2017, 1–10. DOI: 10.1080/10942912.2016.1274904.
- Patil, P.; Mandal, S.; Tomar, S. K.; Anand, S. Food Protein-Derived Bioactive Peptides in Management of Type 2 Diabetes. European. J. Nutr. 2015, 54, 863–880.
- Matsui, T.; Oki, T.; Osajima, Y. Isolation and Identification of Peptidic a-Glucosidase Inhibitors Derived from Sardine Muscle Hydrolyzate. Z. Naturforsch. C Bio. Sci. 1999, 54, 259–263.
- Matsui, T.; Yoshimoto, C.; Osajima, K.; Oki, T.; Osajima, Y. In VitroSurvey ofα-Glucosidase Inhibitory Food Components. Biosci. Biotechnol. Biochem. 2014, 60, 2019–2022. DOI: 10.1271/bbb.60.2019.
- Huang, F. J.; Wu, T. Purification and Characterization of a New Peptide (S-8300) from Shark Liver. J. Food Biochem. 2010, 34, 962–970. DOI: 10.1111/j.1745-4514.2010.00336.x.
- Li-Chan, E. C. Y.; Hunag, S. L.; Jao, C. L.; Ho, K. P.; Hsu, K. C. Peptides Derived from Atlantic Salmon Skin Gelatin as Dipeptidyl-Peptidase IV Inhibitors. J. Agric. Food Chem.. 2012, 60, 973–978. DOI: 10.1021/jf204720q.
- Zhang, Y.; Wang, N.; Wang, W.; Wang, J.; Zhu, Z.; Li, X. Molecular Mechanisms of Novel Peptides from Silkworm Pupae that Inhibit Alpha-Glucosidase. Peptides. 2016, 76, 45–50. DOI: 10.1016/j.peptides.2015.12.004.
- Lee, H. J.; Lee, H. S.; Choi, J. W.; Ra, K. S.; Kim, J. M.; Suh, H. J. Novel Tripeptides with Alpha-Glucosidase Inhibitory Activity Isolated from Silk Cocoon Hydrolysate. J. Agric. Food Chem. 2011, 59, 11522–11525. DOI: 10.1021/jf202686m.
- Yu, Z.; Yin, Y.; Zhao, W.; Liu, J.; Chen, F. Anti-Diabetic Activity Peptides from Albumin against Alpha-Glucosidase and Alpha-Amylase. Food Chem. 2012, 135, 2078–2085. DOI: 10.1016/j.foodchem.2012.06.088.
- Zambrowicz, A.; Timmer, M.; Polanowski, A.; Lubec, G.; Trziszka, T. Manufacturing of Peptides Exhibiting Biological Activity. Amino Acids. 2013, 44, 315–320. DOI: 10.1007/s00726-012-1379-7.
- Zambrowicz, A.; Pokora, M.; Setner, B.; Dabrowska, A.; Szoltysik, M.; Babij, K.; Szewczuk, Z.; Trziszka, T.; Lubec, G.; Chrzanowska, J. Multifunctional Peptides Derived from an Egg Yolk Protein Hydrolysate: Isolation and Characterization. Amino Acids. 2015, 47, 369–380. DOI: 10.1007/s00726-014-1869-x.
- Wang, H.; Du, Y.-J.; Song, H.-C. Α-Glucosidase and Α-Amylase Inhibitory Activities of Guava Leaves. Food Chem. 2010, 123, 6–13. DOI: 10.1016/j.foodchem.2010.03.088.
- Zheng, X. K.; Zhang, L.; Wang, W. W.; Wu, Y. Y.; Zhang, Q. B.; Feng, W. S. Anti-Diabetic Activity and Potential Mechanism of Total Flavonoids of Selaginella Tamariscina (Beauv.) Spring in Rats Induced by High Fat Diet and Low Dose STZ. Journal of Ethnopharmacology. 2011, 137, 662–668. DOI: 10.1016/j.jep.2011.06.018.
- de Azevedo Pereira, R.; Nogueira Batista, J. A.; da Silva, M. C.; Brilhante de Oliveira Neto, O.; Zangrando Figueira, E. L.; Valencia Jimenez, A.; Grossi-de-sa, M. F. An Alpha-Amylase Inhibitor Gene from Phaseolus Coccineus Encodes a Protein with Potential for Control of Coffee Berry Borer (Hypothenemus Hampei). Phytochemistry. 2006, 67, 2009–2016. DOI: 10.1016/j.phytochem.2006.06.029.
- Adler-Nissen, J.;. Determination of the Degree of Hydrolysis of Food Protein Hydrolysates by Trinitrobenzenesulfonic Acid. J. Agric. Food Chem. 1979, 27, 1256–1262. DOI: 10.1021/jf60226a042.
- Taylor, W. H.;. Formol Titration: An Evaluation of Its Various Modifications. Analyst. 1957, 82, 488–498. DOI: 10.1039/an9578200488.
- Sampath Kumar, N. S.; Nazeer, R. A.; Jaiganesh, R. Purification and Identification of Antioxidant Peptides from the Skin Protein Hydrolysate of Two Marine Fishes, Horse Mackerel (Magalaspis Cordyla) and Croaker (Otolithes Ruber). Amino Acids. 2012, 42, 1641–1649. DOI: 10.1007/s00726-011-0858-6.
- Tahir, H. U.; Sarfraz, R. A.; Ashraf, A.; Adil, S. Chemical Composition and Antidiabetic Activity of Essential Oils Obtained from Two Spices Syzygium Aromaticum and Cuminum Cyminum. Int J Food Propert. 2016, 19, 2156–2164. DOI: 10.1080/10942912.2015.1110166.
- Wan, L. S.; Chen, C. P.; Xiao, Z. Q.; Wang, Y. L.; Min, Q. X.; Yue, Y.; Chen, J. In Vitro and in Vivo Anti-Diabetic Activity of Swertia Kouitchensis Extract. J. Ethnopharmacol. 2013, 147, 622–630. DOI: 10.1016/j.jep.2013.03.052.
- Bahadori, M. B.; Dinparast, L.; Zengin, G.; Sarikurkcu, C.; Bahadori, S.; Asghari, B.; Movahhedin, N. Functional Components, Antidiabetic, anti-Alzheimer’s Disease, and Antioxidant Activities of Salvia Syriaca L. Int. J Food Proper. 2016, 1–12. DOI: 10.1080/10942912.2016.1263862.
- Ye, X.-P.; Song, C.-Q.; Yuan, P.; Mao, R.-G. Α-Glucosidase and Α-Amylase Inhibitory Activity of Common Constituents from Traditional Chinese Medicine Used for Diabetes Mellitus. CJNM. 2010, 8, 349–352. DOI: 10.1016/S1875-5364(10)60041-6.
- Mokni Ghribi, A.; Maklouf Gafsi, I.; Sila, A.; Blecker, C.; Danthine, S.; Attia, H.; Bougatef, A.; Besbes, S. Effects of Enzymatic Hydrolysis on Conformational and Functional Properties of Chickpea Protein Isolate. Food Chem. 2015, 187, 322–330. DOI: 10.1016/j.foodchem.2015.04.109.
- Newsholme, P.; Brennan, L.; Bender, K. Amino Acid Metabolism, Beta-Cell Function, and Diabetes. Diabetes. 2006, 55, S39–S47. DOI: 10.2337/db06-S006.
- Salil, G.; Nevin, K. G.; Rajamohan, T. Arginine-Rich Coconut Kernel Diet Influences Nitric Oxide Synthase Activity in Alloxandiabetic Rats. J. Sci. Food. Agr. 2012, 92, 1903–1908. DOI: 10.1002/jsfa.5558.
- Dixon, G.; Nolan, J.; McClenaghan, N.; Flatt, P. R.; Newsholme, P. A Comparative Study of Amino Acid Consumption by Rat Islet Cells and the Clonal Beta-Cell Line BRIN-BD11 - the Functional Significance of L-Alanine. J Endocrinol. 2003, 179, 447–454. DOI: 10.1677/joe.0.1790447.
- Kimball, S. R.; Farrell, P. A.; Jefferson, L. S. Exercise Effects on Muscle Insulin Signaling and Action - Invited Review: Role of Insulin in Translational Control of Protein Synthesis in Skeletal Muscle by Amino Acids or Exercise. J. Appl. Physiol. 2002, 93, 1168–1180. DOI: 10.1152/japplphysiol.00221.2002.
- Matsui, T.; Oki, T.; Osajima, K. Isolation and Identification of Peptidic a-Glucosidase Inhibitors Derived from Sardine Muscle Hydrolyzate. Z. Naturforsch. C Bio. Sci. 1999, 54, 259–263.
- Oseguera-Toledo, M. E.; De Mejia, E. G.; Amaya-Llano, S. L. Hard-To-Cook Bean (Phaseolus Vulgaris L.) Proteins Hydrolyzed by Alcalase and Bromelain Produced Bioactive Peptide Fractions that Inhibit Targets of Type-2 Diabetes and Oxidative Stress. Food. Res. Int. 2015, 76, 839–851. DOI: 10.1016/j.foodres.2015.07.046.